Unveiling the Physics Behind the Exceptional Properties of Iron
Irons extraordinary properties, including high melting point, magnetic susceptibility, and strength, make it a crucial material in various industries.
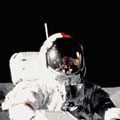
- Anthony Arphan
- 29 min read
Iron, with its impressive strength and durability, has been a cornerstone of human progress for centuries. From constructing massive skyscrapers to forging powerful tools, iron continues to play a vital role in our modern world. But what gives iron its extraordinary properties? How does it possess such a remarkable combination of strength, malleability, and magnetism? The answers lie within the fascinating world of physics.
At the atomic level, iron boasts a unique crystalline structure that contributes to its exceptional strength. The atoms in an iron crystal are arranged in a regular pattern, forming a lattice-like structure. This arrangement allows the atoms to closely bond with each other, creating a strong and rigid material. Furthermore, the presence of interstitial defects within the crystal lattice, such as carbon or nitrogen atoms, can strengthen iron even more by restricting the movement of dislocations – defects that can propagate through the crystal structure and lead to material failure.
The physics behind iron’s strength also lies in its atomic bonding. Iron atoms are bonded together through a metallic bond, which involves the sharing of electrons among a lattice of positively charged iron ions. This bond creates a cohesive force that holds the atoms together, giving iron its characteristic strength. Additionally, the presence of impurities or alloying elements can modify these bonds, resulting in different strength levels and properties.
Besides its strength, iron exhibits remarkable malleability, allowing it to be easily shaped into various forms without breaking. This property is due to the presence of dislocations within its crystal lattice. Dislocations are defects in the lattice structure that allow atoms to move more easily. When a force is applied to iron, these dislocations can move and rearrange, allowing the material to deform and change shape without fracturing. This property makes iron an ideal material for forging and shaping into complex structures.
Aside from its mechanical properties, iron also possesses magnetic properties that have revolutionized technologies such as electromagnetism and modern computing. At the atomic level, the presence of unpaired electrons in iron atoms contributes to its magnetic behavior. These unpaired electrons create tiny magnetic fields, which align themselves in the same direction, resulting in a net magnetic field. This property allows iron to be easily magnetized by an external magnetic field and is the basis for the functioning of magnets and electromagnetic devices.
In conclusion, the extraordinary properties of iron are a product of its unique atomic structure and bonding, which give rise to its strength, malleability, and magnetism. Understanding the physics behind these properties is not only crucial for explaining iron’s role in our everyday lives but also for exploring new possibilities in material science and engineering. The strength of iron is not just a testament to its historical significance, but also a fascinating example of the remarkable forces and interactions at work in the world of physics.
Properties of Iron
Iron is a versatile and incredibly useful metal with a wide range of properties that make it valuable in various industries. Here are some key properties of iron:
1. Strength: Iron is known for its exceptional strength, making it one of the strongest metals available. This property makes it ideal for applications that require high structural integrity and load-bearing capacity.
2. Ductility: Iron is also highly ductile, meaning it can be stretched and bent without breaking. This property allows it to be easily formed into different shapes and structures.
3. Magnetic: One of the most unique properties of iron is its magnetic nature. Iron is a ferromagnetic material, which means it can be magnetized and attracts other magnetic materials.
4. Corrosion Resistance: Iron has excellent corrosion resistance, especially when it is alloyed with other elements like chromium and nickel. This property makes it useful in the production of stainless steel, which is resistant to rust and corrosion.
5. Thermal Conductivity: Iron is a good conductor of heat, allowing it to transfer and distribute heat efficiently. This property makes it important in applications that require heat transfer, such as in cooking utensils and heat exchangers.
6. Electrical Conductivity: While iron is not as good of an electrical conductor as copper or aluminum, it still possesses decent electrical conductivity. This property makes it suitable for electrical wiring and components.
7. High Melting Point: Iron has a high melting point of around 1,538 degrees Celsius (2,800 degrees Fahrenheit), which makes it useful in high-temperature applications like the production of steel and iron casting.
8. Density: Iron is a relatively dense metal with a density of about 7.87 grams per cubic centimeter. This property contributes to its strength and stability.
Overall, iron’s remarkable properties make it a crucial material in numerous industries, including construction, automotive, aerospace, and manufacturing. Without iron and its unique properties, many technological advancements and everyday objects we rely on would not be possible.
Hardness and Durability
Iron is known for its exceptional hardness and durability, which are key factors in its widespread use in various industries. The hardness of iron is a result of its crystalline structure and the presence of impurities. The crystalline structure of iron consists of densely packed atoms, which makes it difficult for external forces to deform or break the metal. This is why iron is often used in the construction of buildings, bridges, and vehicles, where strength and durability are essential.
In addition to its crystalline structure, iron can also be alloyed with other elements to further enhance its hardness. One common alloy is steel, which is an iron alloy that contains small amounts of carbon. The addition of carbon atoms to iron increases its hardness, making it suitable for applications that require resistance to wear and deformation. For example, steel is commonly used in the production of tools, machinery, and structural components.
The durability of iron is closely linked to its hardness. As a hard material, iron is less susceptible to wear and tear, making it ideal for applications that require long-term use. Iron’s durability also stems from its ability to resist corrosion. Iron reacts chemically with oxygen in the presence of moisture to form iron oxide, a process known as rusting. However, the formation of a thin layer of rust on the iron surface acts as a protective barrier against further corrosion, preserving the metal’s integrity.
Iron’s hardness and durability play a crucial role in its many industrial applications. Whether it’s in the construction, automotive, or manufacturing industries, iron’s exceptional properties make it an indispensable material that continues to shape the world around us.
High Melting Point
The high melting point of iron is one of the key factors that contribute to its extraordinary properties. Iron has a melting point of approximately 1,538 degrees Celsius (2,800 degrees Fahrenheit), which is significantly higher than many other metals.
This high melting point is due to the strong metallic bonds present in iron. These bonds involve the sharing of electrons between the iron atoms, creating a lattice structure. The strength of these bonds contributes to the high melting point, as a significant amount of energy is required to break these bonds and convert solid iron into a liquid state.
Iron’s high melting point also makes it suitable for various applications that involve high temperatures. For example, iron is commonly used in the production of steel, which requires the melting and refining of iron ore at extremely high temperatures. Additionally, iron is often used in the construction of engines and other machinery that operate at high temperatures, as it can withstand the heat without losing its structural integrity.
Furthermore, the high melting point of iron allows it to retain its strength and hardness at elevated temperatures. This property is crucial in applications such as aerospace engineering, where materials need to withstand extreme heat conditions without deforming or losing their mechanical properties.
In conclusion, the high melting point of iron is a remarkable characteristic that sets it apart from other metals. It is this property that makes iron invaluable in various industries and applications, contributing to its status as one of the most important and widely used metals in the world.
Magnetic Properties
Iron is known for its exceptional magnetic properties. It has a high magnetic susceptibility, which means it can easily be magnetized. This property is what makes iron a key component in the production of magnets.
When iron is magnetized, it becomes strongly attracted to magnets and gains the ability to attract other magnetic materials. This is due to the alignment of the iron’s electrons, which create a magnetic field around the material.
The magnetic properties of iron are attributed to its atomic structure. Iron has a crystal structure called body-centered cubic (BCC), which consists of closely packed atoms with a high degree of symmetry. This arrangement allows the iron atoms to easily align their spins and create a strong magnetic field.
Iron also has a high Curie temperature, which is the temperature at which it loses its magnetic properties. This temperature is relatively high for iron, around 770°C (1418°F). This means that iron maintains its magnetic properties at typical room temperatures and only loses them at very high temperatures.
The magnetic properties of iron have numerous practical applications. Iron magnets are widely used in everyday devices such as speakers, motors, and generators. Additionally, iron is often used as a core material in transformers to enhance their magnetic field strength.
In summary, iron possesses exceptional magnetic properties due to its high magnetic susceptibility, unique atomic structure, and relatively high Curie temperature. These properties make iron an essential material for the production of magnets and various magnetic devices.
Atomic Structure of Iron
Iron is a metallic element and one of the most abundant elements in the Earth’s crust. It is known for its outstanding strength and durability, which make it a highly useful material in various industries.
At the atomic level, iron has an atomic number of 26, meaning it has 26 protons in its nucleus. It also has 26 electrons, which orbit around the nucleus in different energy levels or shells.
The atomic structure of iron is organized in a hexagonal close-packed (HCP) crystal lattice, which means that the iron atoms are arranged in a compact and closely packed structure. This arrangement allows for the efficient transfer of stress and enhances the material’s strength.
Within the HCP structure, the iron atoms form layers stacked on top of each other. The atoms in each layer are aligned with one another, but the alignment differs between adjacent layers. This structure contributes to the unique properties of iron, such as its high tensile strength and magnetic behavior.
Additionally, the atomic size of iron plays a significant role in its physical properties. The smaller atomic size allows for greater atomic bonding, leading to increased strength and hardness. It also enables iron to exhibit excellent electrical and thermal conductivity.
Understanding the atomic structure of iron is crucial in comprehending its exceptional properties and applications. The arrangement of atoms, bonding, and size all contribute to the overall strength and versatility of this remarkable material.
Iron’s Electron Configuration
Iron, with its atomic number 26, has a unique electron configuration that contributes to its exceptional properties. The electron configuration of an atom describes how its electrons are arranged within the electron shells or energy levels. Understanding iron’s electron configuration is crucial in comprehending its physical and chemical characteristics.
Iron has a total of 26 electrons, distributed among several energy levels. The first energy level, closest to the nucleus, can hold up to two electrons. The second and third energy levels can hold eight electrons each. The fourth energy level, however, can hold a maximum of 18 electrons.
The electron configuration of iron can be abbreviated as [Ar] 3d^6 4s^2. The [Ar] represents the electron configuration of the noble gas argon, which precedes iron in the periodic table. The 3d^6 indicates that there are six electrons in the 3d subshell, while the 4s^2 indicates that there are two electrons in the 4s subshell.
This electron configuration gives iron its unique magnetic properties. The presence of unpaired electrons in the 3d subshell allows iron atoms to align their spins and exhibit ferromagnetism at room temperature. It is this characteristic that makes iron a widely used material in the production of magnets and magnetic devices.
Furthermore, iron’s electron configuration also influences its chemical reactivity. The two valence electrons in the 4s subshell are easily lost or gained, allowing iron to form various compounds with other elements. This versatility in bonding makes iron an essential element in the creation of alloys and diverse chemical materials.
The understanding of iron’s electron configuration provides insights into its remarkable properties and applications in various fields. From its strength in construction to its magnetic behavior, iron’s electron arrangement plays a significant role in defining its role as one of the most useful and abundant elements on Earth.
The Role of Outer Shell Electrons
The extraordinary properties of iron, such as its strength, durability, and ability to conduct heat and electricity, can be attributed to the behavior of its outer shell electrons. Within an iron atom, the outermost shell, known as the valence shell, contains two electrons. These valence electrons play a crucial role in determining the chemical and physical properties of iron.
Iron belongs to the transition metals group, which means its valence shell is not completely filled with electrons. This incomplete valence shell allows iron to form chemical bonds with other elements, leading to the formation of compounds and alloys. The ability of iron to form bonds with other elements is what makes it a versatile material that can be used for various purposes.
Furthermore, the presence of unpaired electrons in the valence shell of iron contributes to its magnetic properties. When a magnetic field is applied, the unpaired electrons align their spins, resulting in a net magnetic moment. This property is what makes iron a commonly used material in magnets and magnetic storage devices.
In addition, the behavior of outer shell electrons also affects the mechanical properties of iron. The interaction between valence electrons and the surrounding lattice structure gives iron its strength and resistance to deformation. The ability of iron to withstand high pressures and retain its shape is crucial for its use in construction and manufacturing industries.
In conclusion, the role of outer shell electrons in iron is pivotal in determining its extraordinary properties. From its chemical reactivity to its magnetic and mechanical properties, the behavior of these electrons greatly contributes to the versatility and usefulness of iron as a material.
Iron’s Crystal Structure
Iron is a metal that possesses a unique crystal structure, which contributes to its exceptional properties. At a microscopic level, the structure of iron is composed of a lattice arrangement of iron atoms, forming a body-centered cubic (BCC) crystal structure.
The BCC crystal structure of iron consists of a regular arrangement of iron atoms, where an iron atom is located at each corner of a cube and another iron atom is located at the center of the cube. This arrangement results in a high packing density and gives iron its strength and toughness.
The lattice structure allows for the movement of dislocations in the crystal, which can help compensate for defects and stresses that occur during deformation. This property is crucial for iron’s ability to withstand extreme forces and retain its shape without fracturing.
Furthermore, the crystal structure of iron plays a significant role in its magnetic properties. Iron is ferromagnetic, meaning it can retain a permanent magnetic field. The BCC crystal structure of iron allows for the alignment of the iron atom’s magnetic moments, resulting in the formation of magnetic domains and the overall magnetization of the material.
Understanding the crystal structure of iron is fundamental to unraveling its extraordinary properties. By studying the arrangement of atoms within the lattice, scientists can gain insights into the strength, toughness, and magnetism of iron, contributing to advancements in materials science and engineering.
Iron’s Molecular Bonds
Iron’s extraordinary properties can be attributed to its unique molecular bonds. In its purest form, iron consists of metallic bonds, which are formed by the delocalization of electrons.
Metallic bonds occur when positively charged metal ions are surrounded by a “sea” of freely moving electrons. These electrons are not confined to any specific atom but are shared among all the metal atoms in the structure. This delocalization of electrons gives iron its high electrical and thermal conductivity, as well as its malleability and ductility.
In addition to metallic bonds, iron also forms covalent bonds. Covalent bonds involve the sharing of electrons between atoms. In the case of iron, covalent bonds are formed with other elements such as carbon, forming compounds like steel.
Iron’s covalent bonds are responsible for its strength and hardness. The shared electrons between iron and carbon atoms create a strong bond, making steel a durable and versatile material. This strength allows iron to withstand high pressures and forces without breaking or deforming.
Furthermore, iron can also form ionic bonds with other elements. Ionic bonds occur when there is a transfer of electrons between atoms, resulting in the formation of ions with opposite charges. This is commonly seen in compounds like rust, where iron reacts with oxygen to form iron oxide.
The combination of metallic, covalent, and ionic bonding in iron makes it a unique and versatile material with a wide range of applications. From construction to manufacturing to transportation, iron’s molecular bonds contribute to its strength, durability, and reliability.
Metallic Bonding in Iron
Iron is a metal that exhibits many remarkable properties, such as its strength and ability to rust. These properties can be attributed to the unique bonding arrangement found in iron, known as metallic bonding.
In metallic bonding, the valence electrons of metal atoms are delocalized and move freely throughout the crystal lattice. This creates a sea of electrons that surround the positively charged metal ions. The metal ions are held together by the attractive forces between their positive charges and the negative charges of the delocalized electrons.
This delocalization of electrons is what gives metals their characteristic properties. The sea of electrons allows metals to conduct electricity and heat very effectively, as the electrons are free to move and carry energy. It also gives metals their malleability and ductility, as the mobile electrons can easily rearrange themselves to accommodate deformation without breaking the atomic bonds.
In the case of iron, metallic bonding is responsible for its high tensile strength. The sea of electrons forms a network of strong metallic bonds that hold the iron atoms together. When a force is applied to iron, the delocalized electrons help distribute the stress throughout the lattice, preventing any localized areas of weakness. This results in the ability of iron to withstand large forces without breaking or deforming.
Furthermore, metallic bonding also plays a role in iron’s ability to rust. When iron comes into contact with oxygen and water, the iron atoms react with the oxygen to form iron oxide. The delocalized electrons in metallic bonding make it easier for oxygen molecules to dissociate and form reactive oxygen ions. These ions can then react with iron atoms, leading to the formation of iron oxide, or rust.
In conclusion, metallic bonding is a key factor in understanding the extraordinary properties of iron. The delocalization of electrons in metallic bonding allows for efficient conduction of electricity and heat, as well as the malleability, ductility, and high tensile strength of iron. It also contributes to the corrosion process in iron. By studying metallic bonding in iron, we can gain a deeper understanding of the physics behind its remarkable properties.
Interatomic Forces in Iron
Iron is a remarkable metal that exhibits extraordinary strength and durability. This can be attributed to the strong interatomic forces present within the material.
The interatomic forces in iron are primarily due to metallic bonding. In metallic bonding, the outermost electrons of each iron atom are delocalized and move freely throughout the crystal lattice. This creates a “sea” of electrons that surround the positively charged iron ions.
These delocalized electrons are responsible for the metallic properties of iron, such as its high thermal and electrical conductivity. They also contribute to its exceptional strength. The movement of the electrons throughout the lattice creates a cohesive force that holds the iron atoms together.
In addition to metallic bonding, there are also weaker interatomic forces known as Van der Waals forces. These forces arise from fluctuations in the electron distribution within iron atoms, leading to temporary dipoles. These temporary dipoles can induce dipoles in neighboring atoms, resulting in attractive forces between them.
Overall, the interatomic forces in iron, including metallic bonding and Van der Waals forces, contribute to its extraordinary properties. Understanding these forces is critical for designing and engineering materials with similar strength and durability.
The Effect of Impurities on Molecular Bonds
Iron is known for its exceptional strength and is widely used in various industries due to its unique properties. However, the presence of impurities in iron can have a significant effect on its molecular bonds, altering its strength and overall performance.
Impurities can be introduced during the manufacturing process or can naturally occur in iron. These impurities can be in the form of other elements or even small clusters of atoms. When impurities are present, they can disrupt the regular arrangement of iron atoms and affect the bonding between them.
One of the main ways impurities impact molecular bonds is through the formation of interstitial sites. These sites are spaces that exist between the regular iron atoms and are occupied by impurity atoms. The presence of impurity atoms in these interstitial sites can weaken or strengthen the iron’s molecular bonds, depending on the nature of the impurity atom.
Impurities can also affect molecular bonds by altering the lattice structure of iron. The lattice structure refers to the arrangement of atoms in a crystal. When impurities are present, they can distort the lattice structure, leading to changes in the bonding forces between iron atoms.
Furthermore, impurities can introduce defects in the crystal lattice, such as dislocations or vacancies. These defects can act as preferential sites for atomic motion and increase the mobility of atoms within the iron. This increased mobility can affect the strength of the molecular bonds and reduce the overall strength of the material.
Overall, the effect of impurities on molecular bonds in iron is complex and dependent on various factors, such as the type and concentration of impurities. Understanding the role of impurities is crucial for controlling the properties of iron and optimizing its performance in different applications.
In conclusion, impurities in iron can have a significant impact on its molecular bonds, affecting its strength and performance. By studying and understanding the effect of impurities, researchers and engineers can develop strategies to minimize their negative effects and enhance the properties of iron.
Strengthening Mechanisms of Iron
Iron is a remarkable metal that possesses extraordinary properties, including its exceptional strength. Understanding the underlying mechanisms that contribute to this strength is crucial in various fields, such as materials science, engineering, and manufacturing.
One of the primary strengthening mechanisms in iron is the presence of impurity atoms, such as carbon, in its crystal lattice. These impurities create lattice distortions and form solid solution alloys, significantly enhancing the strength of iron. The carbon atoms occupy interstitial sites within the iron lattice and effectively prevent the movement of dislocations, which are responsible for plastic deformation.
Another strengthening mechanism is grain size reduction. By refining the grain structure of iron through processes like cold working or heat treatment, the material’s strength can be significantly increased. As the grain size decreases, the number of grain boundaries increases, limiting the motion of dislocations and hindering their ability to propagate. This restriction ultimately leads to higher strength and improved mechanical properties.
The addition of alloying elements, such as manganese, nickel, and chromium, also contributes to the strengthening of iron. These elements form solid solutions or precipitates that obstruct the movement of dislocations, impeding slip and enhancing the material’s resistance to deformation. Furthermore, alloying elements can promote the formation of secondary phases, like precipitates or intermetallic compounds, which provide additional strengthening through dispersion hardening.
Furthermore, strain hardening, also known as work hardening, is another strengthening mechanism of iron. By subjecting the material to plastic deformation, dislocations become entangled and form tangled networks, increasing the resistance to further deformation. This process leads to an increase in strength without significantly altering the chemical composition of the material.
Moreover, heat treatment techniques, such as quenching and tempering, can be utilized to manipulate the strength of iron. Quenching involves rapidly cooling the material from a high temperature, leading to the formation of a hardened microstructure. Tempering, on the other hand, involves reheating the material to a specific temperature and then gradually cooling it. This process improves the material’s toughness while maintaining a desirable level of strength.
In conclusion, the exceptional strength of iron is attributed to various strengthening mechanisms, including impurity atoms, grain size reduction, alloying elements, strain hardening, and heat treatment techniques. Understanding these mechanisms is crucial for designing and manufacturing iron-based materials with superior strength and enhanced performance.
Solid Solution Strengthening
The strength of iron can be significantly enhanced through a process known as solid solution strengthening. This technique involves incorporating another element into the iron crystal lattice structure, creating a solid solution of the two materials.
By introducing the second element, such as carbon or manganese, into the iron lattice, the strength and hardness of the material can be greatly improved. The atoms of the second element occupy the interstitial spaces within the iron lattice and disrupt the regular arrangement of iron atoms.
This disruption creates a distortion in the lattice, making it more difficult for dislocations to move through the material. Dislocations are defects in the crystal lattice structure that can cause plastic deformation and reduce the strength of the material.
As a result, the solid solution strengthening technique increases the resistance of iron to deformation and improves its overall mechanical properties. The strength of iron alloys can be further optimized by carefully controlling the composition and processing conditions.
For example, alloying iron with carbon in certain proportions can create different types of steel, which have varying levels of strength, hardness, and ductility. The addition of other elements, such as chromium or nickel, can also enhance the corrosion resistance of iron alloys.
Solid solution strengthening is a widely used technique in the manufacturing of various iron-based materials, including steels and cast irons. It allows engineers to tailor the properties of these materials for specific applications, such as structural components, automotive parts, and tools.
Grain Size Refinement
Grain size refinement is a crucial factor in determining the strength and mechanical properties of iron. The grain structure of a material refers to the arrangement of individual crystalline grains that make up the solid. In the case of iron, the size of these grains plays a significant role in determining its extraordinary properties.
Larger grain sizes in iron correspond to decreased strength and increased ductility. This is due to the presence of grain boundaries, which are the interfaces between adjacent grains. Grain boundaries act as obstacles to the movement of dislocations, or defects within the crystal structure, leading to enhanced strength.
Refining the grain size of iron can be achieved through various techniques, such as intense plastic deformation or rapid solidification processes. These techniques promote the formation of smaller and more evenly distributed grains, which ultimately enhance the mechanical properties of the material.
The Hall-Petch relationship describes the relationship between grain size and yield strength in crystalline materials. According to this relationship, smaller grain sizes result in higher yield strength.
Grain size refinement also affects other properties of iron, such as hardness, toughness, and fatigue resistance. Smaller grain sizes generally lead to increased hardness and improved fatigue resistance, making the material more resistant to cracking and failure under cyclic loading.
In conclusion, grain size refinement is a critical factor in enhancing the extraordinary properties of iron. By reducing the size of individual grains, the material can exhibit increased strength, hardness, toughness, and fatigue resistance. Understanding the physics behind grain size refinement allows for the development of advanced techniques to optimize the properties of iron and other materials.
Precipitation Hardening
Precipitation hardening, also known as age hardening or solution heat treatment, is a heat treatment technique used to increase the strength and hardness of certain metals, including iron. It involves a sequence of heating and cooling processes that result in the formation of fine particles or precipitates within the metal’s microstructure.
The precipitation hardening process begins with a solution heat treatment, in which the metal is heated to a high temperature to dissolve all the alloying elements into a solid solution. This is followed by a rapid quenching, or cooling, process to cool the metal quickly and prevent the formation of coarse precipitates.
After quenching, the metal is typically aged at a lower temperature for an extended period of time. During this aging process, the previously dissolved alloying elements gradually form small, coherent precipitates within the metal’s microstructure. These precipitates act as obstacles to dislocation movement, making it more difficult for the metal to deform and increasing its strength and hardness.
The time and temperature parameters of the precipitation hardening process are carefully controlled to achieve the desired mechanical properties. Different metals and alloys require specific heat treatment cycles to achieve optimal precipitate formation and distribution.
Precipitation hardening is commonly used in the aerospace and automotive industries, where high-strength and lightweight materials are required. It is also utilized in the production of certain types of steel, aluminum, and titanium alloys, which find applications in structural components, aircraft parts, and sporting goods.
In conclusion, precipitation hardening is a heat treatment technique that enhances the strength and hardness of metals by forming small precipitates within their microstructure. This process plays a crucial role in the development of high-performance materials used in various industrial sectors.
Iron’s Mechanical Properties
Iron is a versatile and widely used metal due to its exceptional mechanical properties. These properties make iron an ideal material for various applications in engineering and construction.
One of the key mechanical properties of iron is its high tensile strength. Tensile strength refers to the ability of a material to resist breaking under tension. Iron has a remarkably high tensile strength, allowing it to withstand large forces without deforming or breaking.
Additionally, iron exhibits excellent ductility, which is the ability of a material to deform under tensile stress without fracturing. This property makes iron suitable for applications that require the material to be shaped or formed into different structures.
Another important mechanical property of iron is its hardness. Hardness is a measure of a material’s resistance to indentation or scratches. Iron is a relatively hard metal, making it resistant to wear and tear in applications that involve friction or contact with other surfaces.
Iron also has a moderate toughness, which is the ability of a material to absorb energy and withstand shock or impact without fracturing. This property makes iron suitable for applications that require the material to endure sudden loads or impacts.
Moreover, iron exhibits good fatigue resistance, which is the ability of a material to resist failure under repeated loading or cycling. This property allows iron structures to withstand repeated stress and strain without experiencing premature failure.
In conclusion, iron possesses exceptional mechanical properties, including high tensile strength, excellent ductility, hardness, moderate toughness, and good fatigue resistance. These properties contribute to iron’s wide range of applications in various industries.
Tensile Strength and Ductility
Tensile strength and ductility are two important properties that define the behavior of iron under stress. Tensile strength refers to the maximum amount of tensile stress that a material can withstand without breaking, while ductility refers to the ability of a material to undergo plastic deformation before breaking.
Iron is known for its exceptional tensile strength, which allows it to withstand large amounts of stress before failing. This is due to the arrangement of iron atoms in its crystal lattice structure, which gives it a strong metallic bonding. The strong metallic bonds between the iron atoms make it difficult for dislocations to move and propagate, resulting in high tensile strength.
However, while iron has high tensile strength, it also exhibits good ductility. Ductility is essential in materials that are prone to undergo deformation under stress, as it allows them to absorb energy before failure. This is particularly important in applications where materials need to be able to deform under stress, such as in structural engineering or manufacturing processes.
The ductility of iron is attributed to its body-centered cubic structure, which provides space for dislocations to move and rearrange under stress. When a tensile force is applied to iron, dislocations start to move and climb through the crystal lattice, allowing the material to undergo plastic deformation. This ability to deform without breaking gives iron its ductility and allows it to be shaped or formed into various useful products.
It is important to note that there is a trade-off between tensile strength and ductility in materials. Typically, materials with high tensile strength tend to have lower ductility, and vice versa. However, iron is able to strike a balance between these properties, making it a highly valuable and versatile material in various industries.
In conclusion, the tensile strength and ductility of iron are key properties that determine its behavior under stress. Iron’s strong metallic bonding and body-centered cubic structure allow it to exhibit both high tensile strength and good ductility, making it an ideal material for applications that require strength and the ability to withstand deformation.
Elastic Modulus
The elastic modulus is a measure of the stiffness or rigidity of a material. It describes how much a material deforms under an applied force and how well it returns to its original shape after the force is removed. In the case of iron, its high elastic modulus contributes to its exceptional strength and durability.
The elastic modulus of iron is one of the highest among commonly used materials. It is a key factor in determining the ability of iron to withstand external forces and maintain its shape. This property is especially important in structural applications, where iron is often used to support heavy loads and resist deformation.
The elastic modulus is influenced by the arrangement of atoms within a material’s crystal lattice. In iron, the atoms are tightly packed and form a regular pattern, allowing the material to exhibit strong interatomic forces and resist deformation.
Iron’s high elastic modulus also contributes to its ability to store and release mechanical energy. When a force is applied to iron, it can deform elastically, storing energy in its atomic bonds. This stored energy can be released when the force is removed, allowing iron to return to its original shape.
The elastic modulus of iron can be quantified using various experimental techniques, such as tensile testing or indentation testing. These tests apply controlled forces to a sample of iron and measure the resulting deformation. By analyzing the relationship between the applied force and the resulting strain, the elastic modulus can be determined.
In conclusion, the high elastic modulus of iron is a crucial factor in its exceptional strength and durability. It allows iron to resist deformation under external forces, maintain its shape, and store and release mechanical energy. Understanding the elastic modulus of iron provides valuable insights into the physics behind its extraordinary properties.
Impact Resistance
The impressive strength of iron is not only attributed to its high tensile strength and hardness, but also its exceptional impact resistance. Impact resistance refers to a material’s ability to withstand sudden or repeated shocks without fracturing or breaking.
Iron’s remarkable impact resistance can be attributed to its unique atomic structure and bonding. The lattice structure of iron consists of closely packed atoms held together by metallic bonds. These metallic bonds enable iron to absorb and distribute the energy caused by impacts, preventing cracks or fractures from forming.
In addition, the presence of impurities and alloying elements in iron can also enhance its impact resistance. The introduction of small amounts of impurities or alloying elements, such as carbon or manganese, can significantly improve the toughness and impact resistance of iron by altering its microstructure.
The impact resistance of iron makes it a crucial material in various applications where toughness and durability are required. For example, in automotive engineering, iron is used to manufacture engine parts, chassis components, and safety structures, as it can withstand the forces caused by collisions and impacts.
Overall, iron’s exceptional impact resistance is a result of its atomic structure, bonding, and the addition of alloying elements. Understanding the physics behind iron’s extraordinary properties can help engineers design more reliable and durable materials for a wide range of applications.