Quantum Computing's Impact on Encryption - A Revolutionary Breakthrough
Quantum Computing and Encryption: Understanding the Impact and Future of Data Security
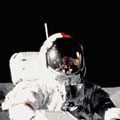
- Anthony Arphan
- 25 min read
In our increasingly connected world, the protection of sensitive information is of utmost importance. With the rise of technology, traditional encryption methods are being challenged by a revolutionary force - quantum technology. By harnessing the power of quantum mechanics, researchers and scientists are delving into the realm of unbreakable codes and decoding complex algorithms. This emerging field has the potential to completely transform the landscape of information security, but at the same time raises concerns about the vulnerability of existing encryption systems.
The beauty of quantum technology lies in its unique ability to manipulate particles in multiple states simultaneously, thanks to the principles of superposition and entanglement. This unparalleled processing power paves the way for the development of quantum computers, which have the potential to solve complex mathematical problems with unprecedented speed. While this may sound like a breakthrough for scientific research and technological advancements, it poses a significant threat to the traditional encryption methods that form the backbone of secure communication and data storage.
Traditional encryption relies on complex algorithms that are time-consuming to crack using classical computers. However, quantum computers have the potential to break down these algorithms at an astonishing rate, rendering traditional encryption methods ineffective. This leaves sensitive information vulnerable to exploitation and unauthorized access. The potential consequences of this are far-reaching, as it could compromise national security, financial systems, and personal privacy.
An Overview of Quantum Computing
In this section, we will provide an insightful glimpse into the world of quantum computation, exploring its immense potential and capabilities. Harnessing the power of quantum mechanics, quantum computing represents a paradigm shift in the realm of information processing. With its ability to exploit the peculiar properties of quantum bits, or qubits, quantum computers offer the promise of solving complex problems that are beyond the reach of classical computers.
Unlike classical computers that rely on binary bits, which can only exist in a state of 0 or 1, quantum computers leverage the superposition and entanglement phenomena of qubits. This allows them to exist in multiple states simultaneously, enabling a quantum computer to perform calculations and process information in ways that defy classical logic and exponentially increase computing power.
One of the key attributes of quantum computing is its potential to revolutionize various fields, including cryptography, optimization, drug discovery, and artificial intelligence. The unique computational abilities of quantum computers give rise to the prospect of breaking conventional encryption algorithms, posing both challenges and opportunities in terms of data security and privacy.
Moreover, quantum computing holds the potential to greatly enhance optimization algorithms, enabling efficient solutions to complex problems such as route planning, portfolio optimization, and supply chain management. Additionally, in fields like drug discovery and material science, quantum computers have the capacity to accelerate simulations and computations, leading to significant advancements in the development of new drugs and materials.
While quantum computing is still in its nascent stages, ongoing research and development efforts are rapidly advancing the field. As technology progresses, the realization of practical quantum computers that can outperform classical computers in specific tasks draws closer. It is important to understand the fundamental concepts and implications of quantum computing in order to fully grasp its potential impact on various aspects of our lives.
The Importance of Encryption
In today’s interconnected digital world, protecting sensitive information has become a paramount concern. The ability to secure data and communication against unauthorized access or interception is crucial for individuals, organizations, and governments alike.
Privacy
Encryption plays a vital role in safeguarding privacy by ensuring that only authorized individuals or entities can access and understand the information being transmitted or stored. It provides a way to keep sensitive data, such as personal details, financial information, and confidential business data, away from prying eyes.
Data Integrity
Encryption not only protects the privacy of information but also ensures its integrity. By encrypting data, any tampering or unauthorized modifications can be detected, as decryption will fail, alerting the intended recipient to the potential compromise.
Trust and Security
Encryption helps establish trust and confidence in digital communication and transactions. When individuals or businesses know that their sensitive data is encrypted, they are more likely to engage in online activities, such as e-commerce, online banking, and teleconferencing, with peace of mind.
Encryption provides a fundamental layer of security that is essential for maintaining privacy, protecting sensitive data, and establishing trust in our increasingly digital world.
Current State of Encryption
With the ever-increasing reliance on digital communication and the storing of sensitive information online, the need for secure encryption has become crucial. Encryption acts as a safeguard, protecting our data from unauthorized access and ensuring privacy. However, advancements in technology and computing power have raised concerns about the effectiveness of current encryption methods.
In today’s digital landscape, encryption algorithms play a fundamental role in securing our data, ranging from personal emails and financial transactions to national security communications. These algorithms employ complex mathematical functions to transform plain text into unintelligible ciphertext, making it virtually impossible for unauthorized individuals to understand the encoded information without the decryption key.
Despite the robustness of current encryption techniques, the rapid development of quantum computing poses a potential threat to their effectiveness. Quantum computers leverage the principles of quantum mechanics to perform calculations significantly faster than classical computers. As a result, they have the potential to break today’s encryption algorithms by quickly factoring large numbers, a fundamental component of many encryption schemes.
The implications of quantum computing on the current state of encryption are still largely theoretical, as practical quantum computers of sufficient power are yet to be realized. However, experts believe that the development of such computers could render many widely-used encryption methods obsolete, potentially compromising the security of sensitive information and communications.
As quantum computing progresses, researchers and experts in the field are actively exploring and developing post-quantum cryptography schemes that can withstand the computational power of quantum computers. These efforts aim to ensure that encryption remains a reliable means of protecting data in a future where quantum computing becomes mainstream.
In conclusion, the current state of encryption is highly effective in safeguarding digital data and privacy. However, the advancements in quantum computing pose a potential challenge to the robustness of existing encryption methods. Ongoing research and the development of post-quantum cryptography offer hope for maintaining secure communication and data protection in the face of emerging technologies.
Classical Computing and Encryption
In the realm of modern computing, the interplay between classical computing and encryption has been a cornerstone of digital security. Classical computing, the conventional approach to processing information, has played a vital role in encryption algorithms, ensuring the confidentiality and integrity of sensitive data.
Encryption serves as a safeguard against unauthorized access to information by transforming it into an unreadable form. It employs complex mathematical algorithms that require specific keys to encrypt and decrypt data. Classical computing techniques have been instrumental in the development and implementation of encryption algorithms, enabling the secure transmission and protection of valuable data.
Conventional computing power has been at the heart of driving encryption techniques, offering computational support for various cryptographic operations. Classical algorithms, such as the Advanced Encryption Standard (AES) and the Rivest-Shamir-Adleman (RSA) algorithm, have been widely used to ensure the confidentiality of sensitive information. These algorithms rely on classical computing capabilities to generate keys, perform mathematical operations, and execute complex encryption and decryption processes.
While quantum computing has garnered significant attention for its potential to break encryption, it is crucial to recognize the invaluable role that classical computing has played in strengthening encryption techniques and establishing digital security. Classical computing continues to serve as a pillar in the realm of encryption, working in tandem with emerging quantum technologies to address the challenges of the future.
Weaknesses of Traditional Encryption
The conventional methods of securing sensitive data through encryption face several vulnerabilities that can be exploited as quantum computing progresses. These weaknesses stem from the limitations of classical encryption algorithms and the evolving nature of threats posed by advancements in technology.
One significant weakness is the reliance on mathematical assumptions, which forms the foundation of traditional encryption. These assumptions, although believed to be secure against current computational power, may prove insufficient against the increased computing capability of quantum computers. As quantum computers employ fundamentally different principles than classical computers, they can potentially undermine the security provided by these assumptions.
Another weakness arises from the inherent vulnerability of encryption keys. Traditional encryption relies on the generation and management of cryptographic keys, which are used to encrypt and decrypt data. However, the security of traditional encryption systems heavily depends on the secrecy and unpredictability of these keys. If an adversary can gain access to the keys through techniques such as brute force attacks, key exchange vulnerabilities, or exploiting weaknesses in key generation algorithms, the encrypted data becomes susceptible to decryption.
Moreover, traditional encryption schemes are often susceptible to attacks that take advantage of mathematical patterns present in encrypted data. These patterns, known as “ciphertext attacks,” can allow attackers to infer certain aspects of the plaintext by examining the encrypted data. While modern encryption algorithms aim to minimize these patterns, the potential power of quantum computing poses a heightened risk, as quantum algorithms can potentially exploit these patterns more efficiently.
In conclusion, the weaknesses of traditional encryption algorithms and systems leave room for vulnerability as quantum computing advances. Understanding these weaknesses is crucial in order to develop new encryption methods that can withstand the emerging threats posed by quantum computers.
The Need for Stronger Security Measures
In today’s rapidly evolving digital landscape, safeguarding sensitive information has become a paramount concern for individuals, organizations, and governments alike. As technology advances, so do the methods employed by malicious actors to exploit vulnerabilities and gain unauthorized access to valuable data. Thus, the urgent need for stronger security measures has arisen to counteract these ever-growing threats.
Ensuring the confidentiality, integrity, and availability of digital information is crucial in protecting personal privacy, maintaining trust in online transactions, and promoting national security. The rapid development of quantum computing poses a significant challenge to traditional encryption algorithms, which are the backbone of modern data security. By harnessing the principles of quantum mechanics, quantum computers have the potential to break conventional encryption methods that currently secure sensitive information.
Consequently, there is a pressing demand for enhanced security measures that can withstand the computational power of quantum computers. These measures must be capable of safeguarding data and communications against quantum attacks, preserving privacy, and maintaining the trustworthiness of cryptographic systems. Without stronger security measures, the integrity of digital infrastructure and the confidentiality of sensitive information could be compromised, leading to dire consequences for individuals, businesses, and nations alike.
Developing and implementing stronger security measures requires collaboration between researchers, industry experts, and policymakers. It entails staying continuously vigilant, investing in research and development of quantum-resistant algorithms, and ensuring the adoption of these new technologies before quantum computing achieves its full potential. Furthermore, it necessitates raising awareness among stakeholders about the urgency of this unprecedented security challenge and taking proactive steps to mitigate any potential risks.
In conclusion, the need for stronger security measures has emerged due to the relentless advancements in technology, including the rise of quantum computing. It is imperative to acknowledge the potential threats posed by quantum computers to existing encryption methods and to actively work towards developing robust, quantum-resistant security systems. Failure to address this need may leave sensitive information vulnerable, compromising privacy, trust, and national security.
Quantum Computing Potential
The revolution brought by quantum technology has the potential to reshape various aspects of our lives, including the field of data security. With the advent of quantum computers, traditional encryption techniques that have long been considered secure face the threat of being compromised. Quantum computers possess unique computational capabilities, allowing them to solve complex problems exponentially faster than classical computers. As such, they hold the potential to break encryption algorithms that protect sensitive information.
Quantum Computers and Their Capabilities
Exploring the realm of quantum technology unveils a new horizon of limitless possibilities. Within this ever-evolving landscape, quantum computers emerge as extraordinary machines that hold immense potential to revolutionize various fields of research and development. Harnessing the principles of quantum mechanics, these advanced computing systems boast unparalleled abilities to solve complex problems that are beyond the scope of classical computers. In this section, we delve into the remarkable capabilities of quantum computers and the groundbreaking advantages they offer.
One of the key aspects that sets quantum computers apart is their ability to rapidly process vast amounts of data. By manipulating quantum bits, or qubits, these machines can perform parallel computations, enabling them to tackle intricate algorithms and calculations more efficiently. Compared to classical computers, quantum computers possess an unmatched capacity to rapidly explore multiple solutions simultaneously, vastly enhancing their problem-solving capabilities.
Another groundbreaking feature lies in the phenomenon of quantum entanglement, a property exclusive to the quantum realm. Quantum entanglement allows qubits to be linked together in such a way that the state of one qubit is inherently connected to the state of another, regardless of the distance between them. This remarkable attribute enables quantum computers to perform highly accurate and precise computations, leading to groundbreaking breakthroughs in domains such as cryptography, optimization, and material science.
Moreover, quantum computers are poised to revolutionize the field of simulation. Their ability to accurately model complex quantum systems holds significant promise for advancing scientific research, creating new insights into molecular structures, chemical reactions, and the behavior of materials at the atomic level. This potential opens up unprecedented opportunities for accelerating drug discovery, designing novel materials, and optimizing energy sources.
- Quantum computers possess an unmatched capacity for parallel computation, enabling them to solve complex problems more efficiently.
- Quantum entanglement allows qubits to be interconnected, leading to highly accurate and precise computations.
- The field of simulation stands to benefit greatly from quantum computing, with accurate modeling of complex quantum systems.
As we continue to push the boundaries of quantum technology, the capabilities of quantum computers are expected to grow exponentially. Although still in its infancy, this rapidly evolving field holds the potential to fundamentally transform industries and reshape our understanding of computation. The future of quantum computers is an exciting prospect, as it promises to tackle challenges that were previously deemed impossible, unlocking new frontiers and breakthroughs in the world of technology.
The Impact on Encryption Algorithms
Exploring the influence of quantum advancements on cryptographic methods.
With the advent of quantum technology, the landscape of encryption algorithms is poised to undergo a significant transformation. The rapid progress in quantum computing poses both unprecedented challenges and promising opportunities for safeguarding sensitive information. This section delves into the impact of quantum computing on encryption algorithms and examines the implications for data security.
Traditional encryption algorithms that have proven resilient against classical computers may no longer be effective in the face of quantum computing’s computational power. As quantum computers leverage the principles of superposition and entanglement to perform calculations at an accelerated pace, conventional encryption methods that rely on factoring large numbers or discrete logarithms may become susceptible to quantum attacks.
One potential consequence of quantum computing’s impact on encryption algorithms is the need for the development and deployment of quantum-resistant encryption techniques. Researchers and cryptography experts have been actively exploring alternative cryptographic approaches, such as lattice-based, code-based, or multivariate cryptography, which are believed to be resistant to quantum attacks. These algorithms rely on mathematical problems that are difficult for both classical and quantum computers to solve, ensuring data security even in the presence of quantum computational capabilities.
Implications | Considerations |
---|---|
1. Vulnerabilities in existing encryption schemes | - Necessity to transition to quantum-resistant algorithms |
2. Protection of sensitive information | - Importance of implementing post-quantum encryption methods |
3. Industry and government impact | - Need for collaboration and standards in adopting new encryption technologies |
As the widespread deployment of quantum computers draws closer, organizations and governments must proactively adapt their encryption strategies to mitigate the potential risks. Transitioning from classical encryption to post-quantum encryption algorithms will be crucial to ensure the continued confidentiality and integrity of sensitive data in a future quantum-powered era.
Potential Risks and Threats
The advent of quantum technology has introduced a new set of challenges and vulnerabilities in the realm of encryption and data security. As we delve into the implications of quantum computing, it becomes apparent that existing encryption algorithms may no longer be able to withstand the power and capabilities of quantum machines.
One potential risk is the compromise of sensitive information due to the ability of quantum computers to quickly and efficiently solve complex mathematical problems that underpin encryption. Traditional encryption methods, such as RSA and AES, rely on mathematical operations that are difficult for classical computers to solve within a reasonable amount of time. However, quantum computers, armed with the power of qubits and the principles of superposition and entanglement, can potentially decipher these cryptographic algorithms with relative ease.
Another threat lies in the potential for quantum computers to retroactively break previously encrypted data. This means that even if data has been encrypted using today’s advanced encryption standards, a future quantum computer could decrypt it in a matter of seconds or minutes, exposing the sensitive information it contains. This poses a significant risk, particularly for long-term confidentiality requirements and sensitive historical data.
Moreover, the arrival of quantum computing poses a challenge for the security of communication systems. Quantum communication protocols, such as quantum key distribution (QKD), provide a means of secure communication by leveraging fundamental principles of quantum mechanics. However, the increased computing power of quantum machines brings the risk of breaking these protocols, potentially compromising the confidentiality and integrity of transmitted data.
Additionally, with the emergence of quantum computing, the landscape of cybersecurity threats could evolve. Malicious actors leveraging quantum technology may be able to develop new attack methods and exploit vulnerabilities that were previously unknown or impractical. This could include the ability to crack encrypted data transmissions, infiltrate secure systems, or even create “time capsules” to store encrypted information until quantum decryption becomes feasible.
Potential Risks | Threats |
---|---|
Vulnerability of existing encryption algorithms | Compromise of sensitive information |
Retroactive decryption of previously encrypted data | Exposure of long-term confidential information |
Challenge to the security of communication systems | Compromised data integrity and confidentiality |
Potential for evolving cyber threats | New attack methods and exploitation of vulnerabilities |
Quantum-Resistant Cryptography
Exploring the Implications of Advanced Computing Technology in the Protection of Sensitive Information
As the world of technology continues to advance at an exponential rate, the need for robust and secure encryption methods becomes increasingly crucial. The rise of quantum computing has sparked both excitement and concern in the realm of cryptography. While quantum computing holds immense potential for solving complex problems at a speed previously unimaginable, it also poses a significant threat to traditional encryption algorithms. In light of this, the concept of quantum-resistant cryptography has emerged as a crucial field of study and development.
Quantum-resistant cryptography refers to the development and implementation of cryptographic techniques that can withstand the challenges posed by quantum computers. Traditional encryption algorithms, such as RSA and AES, rely on mathematical problems that are computationally hard to solve for classical computers. However, quantum computers, with their ability to leverage quantum phenomena like superposition and entanglement, offer the potential to efficiently solve these mathematical problems, rendering current encryption methods obsolete.
The goal of quantum-resistant cryptography is to design encryption algorithms that are resistant to attacks from both classical and quantum computers. This involves exploring alternative mathematical algorithms that are computationally difficult for quantum computers to solve, while still remaining efficient enough for practical implementation. Researchers in the field are actively working towards finding sophisticated cryptographic primitives that can provide long-term security in a post-quantum era.
The development of quantum-resistant cryptography is a multidisciplinary effort that involves researchers from various fields, including mathematics, computer science, and physics. Its importance cannot be overstated, as the widespread adoption of quantum computing threatens the security of sensitive information that underpins modern society. By investing in the research and development of quantum-resistant cryptography, we can stay ahead of malicious actors and ensure that our data remains secure in a future where the power of quantum computing is fully realized.
- Explore the impact of quantum-resistant cryptography on government organizations
- Examine current progress and challenges in developing quantum-resistant algorithms
- Discuss potential applications and use cases of quantum-resistant encryption
- Address the potential timeline for implementing quantum-resistant cryptography
- Highlight the importance of collaboration between academia, industry, and government in ensuring the success of quantum-resistant cryptography
Developing New Encryption Techniques
Exploring Innovative Approaches to Securing Confidential Information
In the ever-evolving landscape of data security, the development of new encryption techniques plays a pivotal role in safeguarding sensitive information from potential breaches. As the field of quantum computing continues to advance, traditional encryption methods are being challenged, necessitating the need for innovative approaches that can mitigate the risks posed by quantum computing.
With the increasing computing power and capabilities of quantum computers, the current encryption protocols may become vulnerable to attacks. As a result, researchers and scientists are actively working on developing alternative encryption techniques that can provide resistance against the computational power of quantum computers. These emerging encryption methods encompass a broad range of algorithms and protocols that utilize unique mathematical concepts and principles.
One approach involves the utilization of lattice-based encryption, which draws on the mathematical properties of lattices. By integrating complex lattice structures within encryption algorithms, it becomes exceedingly difficult for quantum computers to efficiently solve the underlying mathematical problems, thus enhancing security against potential attacks. Lattice-based encryption techniques offer promising avenues for achieving post-quantum security in an increasingly quantum-driven world.
Another avenue being explored is the integration of quantum-resistant cryptography. This approach leverages fundamental principles of quantum mechanics to develop encryption algorithms that are resilient to attacks from both classical and quantum computers. Quantum-resistant cryptography combines the strength of classical cryptographic techniques with the unique properties of quantum systems, providing a robust and secure framework for protecting sensitive data.
Furthermore, ongoing research focuses on the development of quantum key distribution (QKD) protocols. QKD enables the secure exchange of cryptographic keys using quantum mechanics principles. By leveraging quantum phenomena such as entanglement and the uncertainty principle, QKD ensures that any attempted interception of the cryptographic key would disturb the system, alerting the legitimate parties involved. This quantum-resistant method offers a promising solution in guaranteeing secure communication channels.
As the race to develop viable quantum computers advances, so does the urgency to develop new encryption techniques that can withstand the increased computational power they possess. The ongoing research efforts in lattice-based encryption, quantum-resistant cryptography, and quantum key distribution collectively contribute to the development of next-generation encryption methods that strive to ensure the confidentiality and integrity of sensitive information in an increasingly digital world.
Post-Quantum Encryption Standards
The dawn of quantum computing has raised significant concerns about the security of our existing encryption systems. As quantum computers possess tremendous computational power, they could potentially break current encryption algorithms, rendering our sensitive data vulnerable to unauthorized access. To mitigate this risk, researchers and cryptographic experts have been exploring post-quantum encryption standards, which aim to develop new encryption algorithms that can resist attacks from quantum computers.
Post-quantum encryption standards refer to a set of guidelines and regulations that define the requirements and characteristics of cryptographic algorithms that can withstand attacks from quantum computers. These standards encompass various aspects, such as encryption key sizes, algorithmic structures, and resistance to quantum computing-based attacks. The ultimate goal is to ensure the confidentiality, integrity, and authenticity of our sensitive information in a world where quantum computers exist.
The development of post-quantum encryption standards is driven by the urgency to address the impending threat posed by quantum computers to our existing cryptographic infrastructure. As researchers strive to create encryption algorithms that can withstand attacks from quantum computers, they explore different mathematical frameworks, such as lattice-based cryptography, code-based cryptography, multivariate cryptography, and more. These frameworks utilize mathematical problems that are believed to be hard for both classical and quantum computers to solve, ensuring the security of encrypted data against future advances in computing technology.
Standardization bodies, such as the National Institute of Standards and Technology (NIST), are actively involved in the evaluation and selection of post-quantum encryption algorithms. Through open competitions and public reviews, these organizations assess the performance, security, and practicality of different candidate algorithms. The goal is to identify the most promising algorithms that can be adopted as future encryption standards, ensuring the long-term security of our digital communications and sensitive information.
In conclusion, post-quantum encryption standards play a pivotal role in safeguarding our data in the era of quantum computing. By defining guidelines for encryption algorithms that are resilient against attacks from quantum computers, these standards pave the way for a secure future in a rapidly evolving technological landscape.
Implementing Quantum-Resistant Cryptography
In the ever-evolving landscape of information security, the emergence of quantum computing poses a significant threat to the current encryption methods that protect our sensitive data. As quantum computers continue to evolve in their computational power and abilities, traditional encryption algorithms that rely on the difficulty of factoring large integers or solving discrete logarithm problems face the risk of being rendered obsolete.
To address this imminent challenge, researchers and cryptographers have been actively engaged in developing quantum-resistant cryptography, also known as post-quantum cryptography. The objective of quantum-resistant cryptography is to design encryption schemes that can withstand attacks from both classical and quantum computers, ensuring the long-term security of our sensitive information.
The implementation of quantum-resistant cryptography requires a thorough understanding of the underlying principles and mathematical foundations. Instead of relying on mathematical problems that can be efficiently solved by quantum computers, these new encryption schemes explore alternative mathematical structures and computational assumptions that resist attacks from even the most powerful quantum machines.
One approach in implementing quantum-resistant cryptography is through the use of lattice-based encryption schemes. Lattice cryptography relies on the hardness of certain problems related to lattices, which are geometric structures formed by points in multi-dimensional space. These encryption schemes leverage lattice-based algorithms to create secure and efficient key exchange, digital signatures, and encryption algorithms that are resistant to attacks from both classical and quantum adversaries.
Another avenue in developing quantum-resistant cryptography is the exploration of code-based encryption schemes. Code-based cryptography employs error-correcting codes to create encryption schemes resistant to attacks based on the error syndrome decoding problem. These encryption schemes offer a viable solution to quantum-resistant cryptography by utilizing error-correcting codes that are computationally difficult to break, even with the immense power of quantum computers.
As the field of quantum computing progresses, it is crucial to invest in research and development of quantum-resistant cryptography to ensure the security and privacy of our digital world. By implementing robust encryption schemes that can withstand attacks from quantum adversaries, we can mitigate the risks associated with the potential decryption of sensitive information and maintain the confidentiality of our data in the quantum era.
Challenges and Considerations
The task of harnessing the powerful potential of quantum technology in the realm of encryption presents a multitude of unique challenges and considerations. As we explore the impact of quantum computing in breaking encryption mechanisms, certain aspects come to the forefront that demand careful analysis and strategic planning.
First and foremost, the emergence of quantum computing introduces a paradigm shift in the very fabric of traditional encryption algorithms. The conventional approach to securing sensitive data through encryption may no longer be sufficient to protect against the computational prowess of quantum machines. This necessitates a comprehensive reassessment of existing encryption protocols and a search for quantum-resistant alternatives.
Furthermore, the transition from classical to quantum encryption methods engenders a range of practical challenges. Quantum systems are highly sensitive to noise, interference, and errors, demanding sophisticated error correction techniques to maintain the integrity and accuracy of quantum computations. As such, the development and implementation of robust error-correction algorithms become imperative to ensure the reliability and security of quantum encryption systems.
Another critical consideration lies in the sheer scale of the computational resources required for effective quantum encryption measures. Quantum computers encompass a vast number of qubits, exponentially surpassing the capabilities of classical computers. This necessitates a substantial investment in developing not just more powerful quantum hardware, but also the necessary infrastructure to support the intricate requirements of quantum encryption protocols.
Add to these challenges the inherent difficulties in developing and standardizing quantum-resistant encryption algorithms, and it becomes evident that the road to establishing robust quantum encryption solutions is riddled with obstacles. Collaborative efforts among scientists, mathematicians, and industry experts become essential to address these challenges collectively and strive for a secure and resilient encryption landscape in the quantum era.
Limitations and Practical Implications
In exploring the subject of quantum computing’s impact on encryption, it is important to consider the inherent limitations and practical implications that arise from this emerging technology. By delving into the potential challenges and tangible consequences, we can gain a deeper understanding of the complexities involved.
One key limitation to be aware of is the potentially high cost associated with quantum computing. This advanced field of scientific research requires significant financial investment in terms of hardware, infrastructure, and skilled personnel. Moreover, the computational power needed for quantum computing remains a practical challenge, as quantum computers are currently quite limited in their functionality.
Furthermore, the practical implications of quantum computing on encryption systems cannot be overlooked. Traditional encryption algorithms, which are used to secure sensitive data and communications, may become vulnerable to attack when faced with the immense computing power of quantum computers. This presents a pressing concern for industries that rely heavily on encryption for confidentiality, such as banking, government, and healthcare.
Additionally, the widespread adoption and implementation of quantum-resistant encryption methods will require significant effort and coordination. Updating existing systems and protocols to be compatible with quantum-resistant algorithms is a complex task, requiring extensive research, testing, and standardization. The transition to quantum-safe encryption will not be instantaneous and may prove to be a lengthy and resource-intensive process.
In conclusion, the limitations and practical implications of quantum computing in the context of encryption pose substantial challenges to the current state of cybersecurity. While quantum computing holds great promise for advancing various fields, it also necessitates careful consideration and proactive measures to ensure the robustness of encryption systems in the face of this rapidly progressing technology.
Transitioning to Quantum-Resistant Cryptography
In the rapidly evolving landscape of cryptographic security, the emergence of quantum computing poses a significant threat to traditional encryption algorithms. With the potential to break conventional encryption methods, quantum computers have raised concerns about the security of sensitive data in various sectors. This section focuses on the essential concept of transitioning to quantum-resistant cryptography as a proactive measure against the future risks of quantum computing.
The transition process also necessitates collaboration between various stakeholders, including cryptographic experts, software developers, and industry regulators. Their collective efforts are vital in developing standardized quantum-resistant cryptographic algorithms and protocols, ensuring interoperability and widespread adoption across different systems and platforms.
Furthermore, transitioning to quantum-resistant cryptography demands a continuous enhancement of knowledge and skills in cryptographic techniques. Organizations need to invest in research and development to stay abreast of the latest advancements in quantum-resistant algorithms and ensure their implementation is both efficient and effective.
In conclusion, the transition to quantum-resistant cryptography presents an essential step in safeguarding sensitive data in an era where quantum computing could potentially compromise traditional encryption methods. By embracing this proactive measure and collaborating with experts across the field, organizations can ensure the resilience of their cryptographic systems against the ever-evolving threat landscape.