Exploring the Connection Between Iron and Alzheimer's Disease - Decoding the Enigma
Iron and Alzheimers Disease: Understanding the Complex Relationship Between Iron Dysregulation and Neurodegeneration
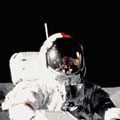
- Anthony Arphan
- 26 min read
In the quest to peel back the layers of mystery surrounding Alzheimer’s disease, scientists have turned their attention to a previously unexplored connection - the intricate alliance between iron and the perplexing malady that affects millions worldwide. Armed with an insatiable curiosity and an insatiable hunger for answers, researchers have embarked on a journey to unravel the enigma that lies within the peculiar nexus of iron and Alzheimer’s disease. With each piece of the puzzle slowly falling into place, a clearer picture emerges, unveiling a link that is both subtle and yet undeniably profound.
Within the labyrinthine corridors of the human brain, iron assumes a role that stretches far beyond its reputation as a mere mineral. Often associated with strength and resilience, iron’s presence within the brain hints at a complex tale intricately woven. Its involvement in vital physiological processes, such as oxygen transport and neurotransmitter regulation, establishes it as a crucial element of neural function. However, recent evidence has unveiled a dark side to this indispensable component, as iron’s alluring embrace intertwines with the progression of Alzheimer’s disease in unexpected ways.
The unforeseen complexities of this partnership between iron and Alzheimer’s disease lie in the delicate balance between necessity and toxicity. On one hand, iron’s integral role in the brain is undeniable, as it supports the growth and maintenance of neurons and synapses. However, like a double-edged sword, excessive iron levels can unleash a cascade of detrimental effects. The intricate interplay between iron and the pathological hallmarks of Alzheimer’s disease - amyloid plaques and tau tangles - further compound the mystery, fueling speculation of an intricate relationship that is begging to be decoded.
Iron Accumulation in the Brain
The existence of an intriguing relationship between iron levels and Alzheimer’s disease has captured the attention of researchers in recent years. A growing body of evidence suggests that there is a direct association between the accumulation of iron in the brain and the onset and progression of this neurodegenerative disorder.
Iron deposition in the brain refers to the abnormal build-up of iron within specific regions, disrupting normal functioning and triggering a cascade of detrimental effects. This phenomenon has been observed in various brain regions, including the hippocampus, basal ganglia, and cerebral cortex.
Cautionary studies have established that excessive iron accumulation can adversely impact neuronal health, leading to neuronal death, impairments in synaptic plasticity, and the formation of toxic protein aggregates. Additionally, iron accumulation has been linked to increased oxidative stress and inflammation, which are believed to exacerbate neurodegenerative processes.
Iron dyshomeostasis, characterized by an imbalance in iron regulation, has emerged as a potential culprit in the development of Alzheimer’s disease. Disruption in iron metabolism can disrupt the delicate equilibrium between iron transport, storage, and utilization in the brain, eventually culminating in the accumulation of iron and subsequent neuronal damage.
Elucidating the mechanisms underlying iron accumulation in the brain is of paramount importance to shed light on the pathogenesis of Alzheimer’s disease and explore potential therapeutic targets. Future research endeavors are focused on unraveling the intricate interplay between iron and the multiple molecular pathways implicated in the disease, ultimately aiming to develop preventive and therapeutic strategies to combat this debilitating disorder.
The Role of Iron in the Body
In the intricate web of human biology, iron maintains a vital position through its multifaceted contributions. Found abundantly in every cell, this essential mineral facilitates the transport of oxygen throughout the body, fuels energy production, and supports proper immune function. Iron serves as a cornerstone for red blood cells, ensuring that they effectively deliver oxygen to tissues and organs. Furthermore, it plays a pivotal role in enzyme activity, DNA synthesis, and cellular respiration.
Oxygen Transportation and Energy Production: Without iron, the body’s ability to transport oxygen and generate energy would be greatly impaired. Hemoglobin, the protein responsible for carrying oxygen in red blood cells, relies on iron to bind and transport this life-sustaining gas. By facilitating oxygen delivery to tissues, iron allows cells to produce adenosine triphosphate (ATP), the main energy currency of the body. Iron is also involved in the metabolism of carbohydrates, fats, and proteins, contributing to optimal energy production.
Immune Function: Iron’s influence extends beyond oxygen transportation and energy production, playing a critical role in maintaining a robust immune system. Iron supports the proper function of immune cells by aiding the production of antibodies and the activation of certain immune responses. On the other hand, iron balance is crucial in preventing microbial growth, as excess iron can fuel the replication of certain pathogens. Balancing iron levels is essential to ensure an effective immune response while avoiding potential harm.
Enzyme Activity and Cellular Health: Within cells, iron takes part in various enzymatic reactions essential for the body’s overall health. Many enzymes involved in DNA synthesis, repair, and cell division require iron as a cofactor. Iron’s presence assists in the synthesis of collagen, a crucial protein in connective tissues, and supports the function of enzymes involved in neurotransmitter production. Furthermore, iron aids in maintaining cellular respiration, enabling efficient energy production within mitochondria. Overall, iron’s involvement in enzyme activity is essential for maintaining the body’s structural integrity and efficient cellular functioning.
Understanding the extensive role of iron in the body highlights its significance beyond its association with Alzheimer’s disease. From oxygen transportation to enzyme activity, iron is an indispensable component in maintaining overall health and wellbeing.
Iron Metabolism in the Brain
The intricate relationship between iron and the development of Alzheimer’s disease has long intrigued researchers in their quest to unravel the enigma. In the context of the brain, the process of iron metabolism plays a crucial role in maintaining its delicate equilibrium.
Iron, an essential element required for various physiological processes, including oxygen transport and energy production, participates in intricate metabolic pathways within the brain. The precise balance of iron levels is essential for proper brain function, as both iron deficiency and iron overload have been associated with detrimental effects on cognitive health.
Iron metabolism in the brain involves multiple mechanisms, including iron acquisition, storage, and transport. Proteins such as transferrin and ferritin regulate the levels of iron in the brain, ensuring its availability when needed and preventing excessive accumulation that can lead to neurodegenerative disorders like Alzheimer’s disease.
Iron acquisition in the brain occurs through the blood-brain barrier, a semipermeable membrane that tightly regulates the entry of substances into the brain. Specialized transport proteins facilitate the movement of iron into the brain cells, where it is utilized for various cellular processes.
Once inside the brain cells, iron is stored within ferritin, a protein complex that acts as a reservoir for iron. Ferritin not only regulates iron levels but also acts as a protective mechanism against iron-induced oxidative damage, which is implicated in the progression of Alzheimer’s disease.
Proper iron transport within the brain is crucial for maintaining the balance between iron availability and storage. The iron-binding protein transferrin binds to iron, allowing it to be transported across cell membranes and delivered to specific regions of the brain where it is required for cellular functions.
The intricate network of iron metabolism in the brain highlights its indispensable role in maintaining cognitive health. Disruptions in this delicate balance can lead to the accumulation of iron and subsequent oxidative stress, contributing to the pathogenesis of neurodegenerative diseases like Alzheimer’s. Understanding the mechanisms that regulate iron metabolism in the brain is crucial for developing potential therapeutic strategies to combat Alzheimer’s disease and related disorders.
Mechanisms of Iron Accumulation in Alzheimer’s Disease
Understanding the mechanisms behind the accumulation of iron in Alzheimer’s disease is crucial in unraveling the complexities of this neurodegenerative disorder. By examining the processes through which iron builds up in the brain of individuals with Alzheimer’s disease, researchers aim to shed light on the underlying causes and potential therapeutic targets.
Iron, a crucial element for various physiological processes, becomes imbalanced in the brains of individuals with Alzheimer’s disease. This dysregulation of iron homeostasis leads to the accumulation of iron in specific regions of the brain, such as the hippocampus and cerebral cortex. The exact mechanisms through which iron accumulates remain a subject of ongoing research, but several possible pathways have emerged.
Oxidative stress, a state of imbalance between antioxidant defenses and reactive oxygen species, plays a significant role in iron accumulation. Increased oxidative stress in the brain can disrupt iron metabolism, leading to the buildup of iron in neuronal tissues. Moreover, alterations in iron regulatory proteins and transporters, such as transferrin and ferritin, may contribute to the abnormal retention of iron in Alzheimer’s disease.
The involvement of amyloid beta, a protein fragment that aggregates to form the hallmark plaques in Alzheimer’s disease, has been linked to iron accumulation. Amyloid beta can bind to iron ions, facilitating their deposition and ultimately leading to the accumulation of iron in the brain. Additionally, interactions between iron and other molecules involved in Alzheimer’s disease pathology, such as tau protein, further contribute to iron accumulation and neurotoxicity.
Emerging evidence also suggests a role for inflammation in iron accumulation. Chronic inflammation in the brain, driven by factors such as activated microglia and cytokines, can disrupt iron regulation and promote its accumulation. In turn, iron accumulation can exacerbate inflammation, establishing a vicious cycle that fuels the progression of Alzheimer’s disease.
In conclusion, understanding the mechanisms underlying iron accumulation in Alzheimer’s disease is essential for developing targeted therapeutic interventions. Oxidative stress, alterations in iron regulatory proteins, amyloid beta, and inflammation are all implicated in the dysregulation of iron homeostasis. Further research is required to unravel the intricate interplay among these mechanisms and identify potential avenues for intervention in preventing or slowing down the progression of Alzheimer’s disease.
Iron-Induced Oxidative Stress
Oxidative stress caused by an excess of iron in the body has emerged as a significant factor in the development and progression of Alzheimer’s disease. The connection between iron levels and the pathogenesis of this neurodegenerative disorder has been a subject of extensive research. Understanding the mechanisms by which iron induces oxidative stress can provide insights into potential therapeutic targets for Alzheimer’s disease.
Excessive iron accumulation in the brain can lead to a disruption in the delicate balance of antioxidants and reactive oxygen species (ROS). This imbalance triggers a cascade of events that result in oxidative stress, a harmful condition characterized by the production of free radicals that damage cellular components. The brain, with its high metabolic activity and abundance of unsaturated lipids, is particularly vulnerable to oxidative damage.
- The role of iron in the generation of ROS: Iron, through its ability to cycle between its ferrous (Fe²⁺) and ferric (Fe³⁺) states, can participate in Fenton and Haber-Weiss reactions, resulting in the production of highly reactive hydroxyl radicals. These hydroxyl radicals, known as the “most damaging free radicals,” can initiate a chain reaction of lipid peroxidation, protein oxidation, and DNA damage, leading to cellular dysfunction and neurodegeneration.
- Iron-induced neuroinflammation: Elevated iron levels can trigger neuroinflammatory responses, amplifying the oxidative stress cascade. Activated microglia, the resident immune cells in the brain, release pro-inflammatory cytokines and generate ROS, further exacerbating the oxidative damage. Chronic inflammation, as a consequence of iron-induced oxidative stress, contributes to the progression of Alzheimer’s disease.
- Iron dysregulation and amyloid-beta pathology: Accumulation of amyloid-beta plaques, another hallmark of Alzheimer’s disease, is closely linked to iron dysregulation. Iron can promote the aggregation of amyloid-beta peptides, which not only exacerbates oxidative stress but also contributes to the formation of neurofibrillary tangles and neuronal dysfunction.
Understanding the intricate relationship between iron-induced oxidative stress and the pathogenesis of Alzheimer’s disease can provide a foundation for the development of therapeutic strategies aiming to modulate iron levels, enhance antioxidant defenses, and attenuate neuroinflammation. Further research focused on unraveling this mystery may pave the way for novel interventions in the prevention and treatment of Alzheimer’s disease.
Reactive Oxygen Species and Oxidative Damage
Exploring the Relationship between Reactive Oxygen Species (ROS) and the detrimental effects of Oxidative Damage unveils a significant aspect of the intricate puzzle surrounding the connection of Iron and Alzheimer’s disease. By examining the role of ROS and its impact on cellular structures, we can shed light on the mechanisms underlying the pathogenesis of this neurodegenerative disorder.
ROS refers to chemically reactive molecules containing oxygen, formed as natural byproducts of various cellular metabolic processes. These highly reactive species can cause oxidative damage to lipids, proteins, and nucleic acids within cells. Oxidative damage, in turn, involves the structural and functional impairment resulting from the excessive production or insufficient removal of ROS.
Understanding the interplay between ROS and oxidative damage is crucial in unraveling the complexities of Alzheimer’s disease. Elevated levels of ROS can trigger a cascade of events that contribute to the formation of toxic protein aggregates, such as beta-amyloid plaques and neurofibrillary tangles, which are characteristic pathological hallmarks of the disease.
Furthermore, oxidative damage caused by ROS can disrupt normal cellular processes, leading to mitochondrial dysfunction, impaired synaptic plasticity, and neuronal death. This disruption contributes to the progressive cognitive decline and memory impairment observed in Alzheimer’s patients.
Investigating the extent of ROS-induced oxidative damage and elucidating the underlying mechanisms can potentially guide the development of targeted therapeutic strategies. By discovering ways to modulate ROS production or enhance cellular antioxidant defense mechanisms, we may be able to mitigate the harmful effects of oxidative stress and potentially slow down the onset and progression of Alzheimer’s disease.
Iron as a Catalyst for Oxidative Stress
Oxidative stress, a significant factor in the development of various neurodegenerative diseases, has been associated with the role of iron as a catalyst. Iron, an essential nutrient for proper bodily functions, can become a double-edged sword when its levels exceed the body’s requirements.
When iron is present in excessive amounts or is not regulated properly, it can promote the production of reactive oxygen species (ROS), leading to oxidative stress. ROS are highly reactive molecules that can damage cellular components such as proteins, lipids, and DNA, contributing to the development and progression of neurodegenerative diseases.
- Iron overload can occur due to various factors, including genetic predisposition, dietary intake, and impaired iron metabolism. This excess iron can accumulate in the brain, particularly in regions affected by Alzheimer’s disease, potentially exacerbating oxidative stress.
- Moreover, iron has been found to interact with amyloid-beta, a protein fragment that forms plaques in the brains of individuals with Alzheimer’s disease. This interaction further enhances oxidative stress and contributes to the progression of the disease.
- Furthermore, iron-mediated oxidative stress can disrupt normal cellular processes, impair mitochondrial function, and cause neuronal damage. This damage can lead to the development of cognitive impairments and other symptoms associated with Alzheimer’s disease.
- Understanding the intricate relationship between iron and oxidative stress is crucial for developing potential therapeutic interventions targeting neurodegenerative diseases. Strategies aimed at regulating iron levels, minimizing iron-mediated oxidative stress, and promoting antioxidant defenses may help mitigate the progression of Alzheimer’s disease and other related disorders.
Overall, the role of iron as a catalyst for oxidative stress underscores its potential significance in the pathogenesis of Alzheimer’s disease. Modulating iron levels and minimizing iron-induced oxidative stress could present new avenues for therapeutic interventions and the prevention of neurodegenerative diseases.
Implications of Oxidative Stress in Alzheimer’s Disease
Oxidative stress is a significant factor in the development and progression of Alzheimer’s disease. It plays a crucial role in the cellular damage and dysfunction observed in the brain of individuals with this neurodegenerative disorder.
Excessive production of reactive oxygen species (ROS) and impaired antioxidant defense mechanisms lead to an imbalance in the redox state of the brain. This imbalance results in oxidative stress, causing oxidative damage to lipids, proteins, and nucleic acids.
The detrimental effects of oxidative stress in Alzheimer’s disease are multifaceted. Firstly, oxidative damage disrupts neuronal cell membranes, leading to impaired cell signaling and communication. It also contributes to the formation of amyloid plaques and neurofibrillary tangles, the pathological hallmarks of Alzheimer’s.
Moreover, oxidative stress further exacerbates neuroinflammation, which amplifies the neurodegenerative process. Dysregulation of the immune response and the release of pro-inflammatory cytokines contribute to the destruction of neurons and synaptic dysfunction.
Furthermore, oxidative stress affects mitochondrial function, leading to energy metabolism impairment and increased production of ROS. This vicious cycle perpetuates oxidative stress and further damages neuronal cells.
Understanding the implications of oxidative stress in Alzheimer’s disease is of utmost importance for the development of effective therapeutic strategies. Targeting oxidative stress pathways and enhancing antioxidant defenses could help mitigate the progression of neuronal damage and potentially delay the onset of symptoms.
Iron and Beta-Amyloid Accumulation
The relationship between iron levels and the build-up of beta-amyloid plaques in the brain is a topic of growing interest and investigation in the field of Alzheimer’s disease research. Understanding the connection between iron and beta-amyloid accumulation could provide valuable insights into the underlying mechanisms of the disease and potentially lead to new therapeutic approaches.
Iron, an essential nutrient for brain function, plays a vital role in various neurological processes. However, an imbalance in iron levels can lead to oxidative stress and the production of reactive oxygen species, which have been implicated in the development of Alzheimer’s disease. Accumulation of beta-amyloid, another hallmark characteristic of Alzheimer’s, has also been linked to oxidative stress. The question arises: could there be a link between iron and beta-amyloid accumulation?
Emerging research suggests that iron overload in the brain may promote the aggregation and accumulation of beta-amyloid plaques. Studies have found elevated levels of iron in the brains of individuals with Alzheimer’s, particularly in areas associated with beta-amyloid deposition. Furthermore, iron has been shown to interact with beta-amyloid peptides, promoting their aggregation into toxic forms that contribute to the progression of the disease.
Iron accumulation and beta-amyloid deposition create a vicious cycle, as the presence of beta-amyloid can disrupt iron homeostasis and further contribute to iron overload. This interplay between iron and beta-amyloid may contribute to the neuronal dysfunction, neuroinflammation, and oxidative damage observed in Alzheimer’s disease.
Understanding the mechanisms underlying iron and beta-amyloid accumulation is a complex puzzle that requires further exploration. However, the potential significance of this relationship cannot be underestimated. Finding ways to modulate iron levels and prevent or reverse beta-amyloid accumulation could offer new avenues for the development of effective treatments for Alzheimer’s disease.
- Iron imbalance and beta-amyloid aggregation: a significant connection
- Elevated iron levels in the brains of Alzheimer’s patients
- The interaction between iron and beta-amyloid: promoting toxicity
- The vicious cycle of iron overload and beta-amyloid deposition
- Unraveling the mystery: uncovering the mechanisms of iron and beta-amyloid in Alzheimer’s disease
The Role of Beta-Amyloid in Alzheimer’s Disease
In the field of Alzheimer’s disease research, one significant area of focus is understanding the role that beta-amyloid plays in the development and progression of this condition. Beta-amyloid, a protein fragment, is believed to have a connection to the onset and progression of Alzheimer’s disease. Exploring the functions and effects of beta-amyloid in the brain is crucial for gaining insights into the underlying mechanisms of this debilitating neurodegenerative disorder.
Researchers have put forth various hypotheses regarding the specific role of beta-amyloid in Alzheimer’s disease. One prominent theory suggests that an accumulation of beta-amyloid plaques disrupts the communication between nerve cells, leading to impaired cognitive functions and memory loss. This hypothesis proposes that beta-amyloid acts as a toxic agent, triggering a chain of events that ultimately leads to the characteristic symptoms of Alzheimer’s disease.
Another perspective focuses on the potential role of beta-amyloid in the formation of neurofibrillary tangles, which are abnormal twisted fibers found within the brain cells of individuals with Alzheimer’s disease. Studies suggest that beta-amyloid contributes to the formation of these tangles, which disrupt the normal functioning of neurons and contribute to the degeneration of brain tissue.
Moreover, some researchers hypothesize that beta-amyloid may also interact with other substances in the brain, such as metals like iron, copper, and zinc. This interaction could further exacerbate the damaging effects of beta-amyloid and contribute to the progression of Alzheimer’s disease. Understanding the interaction between beta-amyloid and these metals is crucial for uncovering potential therapeutic targets and interventions.
While the exact role of beta-amyloid in Alzheimer’s disease is still a subject of ongoing research and debate, its involvement in the pathogenesis of this condition highlights the importance of studying and understanding its functions. By unraveling the mysteries surrounding beta-amyloid, scientists hope to develop effective treatments and preventive strategies that could mitigate the impact of Alzheimer’s disease on affected individuals and their families.
Iron as a Modulator of Beta-Amyloid Aggregation
In the ongoing exploration of the intricate relationship between iron and Alzheimer’s disease, researchers have turned their attention to the role of iron in modulating the aggregation of beta-amyloid peptides. This emerging field of study aims to unravel the underlying mechanisms by which iron influences the formation and progression of beta-amyloid plaques, a hallmark feature of Alzheimer’s disease.
Understanding the impact of iron on beta-amyloid aggregation has the potential to shed light on new therapeutic strategies for Alzheimer’s disease. Beta-amyloid is a peptide that spontaneously aggregates into insoluble fibrils, which are the primary constituents of amyloid plaques found in the brains of Alzheimer’s patients. Iron, as an essential element for cellular processes, has been shown to promote or inhibit the aggregation of beta-amyloid, depending on its concentration and redox state.
Studies have revealed that excessive iron levels in the brain can accelerate the aggregation of beta-amyloid, leading to the formation of more toxic and insoluble aggregates. Conversely, iron chelation therapies, which aim to reduce the iron levels in the brain, have shown promising results in inhibiting beta-amyloid aggregation and preventing the formation of amyloid plaques. These findings highlight the potential for targeting iron as a therapeutic approach to prevent or slow down the progression of Alzheimer’s disease.
Further investigations into the molecular mechanisms underlying the influence of iron on beta-amyloid aggregation are still needed. Researchers are focusing on elucidating the specific interactions between iron and beta-amyloid, as well as the pathways through which iron influences the aggregation process. By gaining a deeper understanding of these fundamental mechanisms, scientists hope to identify potential drug targets that can modulate iron levels or its interaction with beta-amyloid, thereby preventing or reversing the pathological aggregation that characterizes Alzheimer’s disease.
Interplay Between Iron and Beta-Amyloid in Alzheimer’s Pathology
The intricate relationship between iron and beta-amyloid presents a significant focus of research in understanding the underlying mechanisms of Alzheimer’s disease. By exploring the interplay between these two factors, researchers aim to unravel the complex web of pathology that contributes to the development and progression of this neurodegenerative disorder.
The presence of excessive iron levels within the brain has been consistently associated with Alzheimer’s pathology. Iron, a critical element for various biological processes, can accumulate and become dysregulated in the brain, leading to oxidative stress and neurotoxicity. Beta-amyloid, on the other hand, is a peptide that forms sticky plaques in the brain, another hallmark of Alzheimer’s disease. Emerging evidence indicates that iron and beta-amyloid mutually influence each other, potentially amplifying their detrimental effects on neuronal function and integrity.
Iron has been shown to promote the aggregation and accumulation of beta-amyloid, exacerbating the formation of toxic plaques. Conversely, beta-amyloid can bind and sequester iron, disrupting its normal cellular distribution and potentially leading to further iron dysregulation. This bidirectional relationship between iron and beta-amyloid highlights a complex interplay that may contribute to the progression and severity of Alzheimer’s disease.
Furthermore, iron has been implicated in the generation of reactive oxygen species (ROS), which contribute to oxidative damage in the brain. The interaction between iron and beta-amyloid can enhance ROS production, leading to increased oxidative stress and neuronal injury. This interplay may provide insights into the mechanisms underlying the neurodegenerative processes observed in Alzheimer’s disease.
Understanding the interdependence of iron and beta-amyloid in Alzheimer’s pathology is crucial for the development of targeted therapeutic interventions. By elucidating the complex relationship between these factors, researchers can potentially identify novel therapeutic targets and interventions aimed at modulating iron levels and beta-amyloid accumulation, thereby mitigating the impact of Alzheimer’s disease.
Iron Dysregulation and Neuroinflammation
Exploring the intricate connection between iron dysregulation and neuroinflammation sheds light on an intriguing aspect of the complex relationship between Alzheimer’s disease and iron levels in the brain.
Iron plays a vital role in numerous physiological processes within the body, including oxygen transport, energy production, and DNA synthesis. However, in the context of neuroinflammation, imbalances in iron homeostasis can have detrimental effects on neuronal function and overall brain health.
- The disruption of iron regulation pathways has been implicated as a contributing factor to the development and progression of neuroinflammation in Alzheimer’s disease.
- Elevated iron levels can lead to the generation of reactive oxygen species (ROS), initiating a cascade of inflammatory responses that can damage neurons and promote neurodegeneration.
- Conversely, iron deficiency in the brain can impair crucial processes such as myelination and neurotransmitter synthesis, impacting neuronal communication and contributing to neuroinflammatory states.
Understanding the interplay between iron dysregulation and neuroinflammation holds promise for the development of potential therapeutic interventions that target these processes to mitigate the progression of Alzheimer’s disease.
The Role of Inflammation in Alzheimer’s Disease
Inflammation has emerged as a significant factor in the development and progression of Alzheimer’s disease, shedding light on the intricate interplay between the immune system and neurological disorders. The activation of inflammatory processes in the brain can have detrimental effects on neuronal health, leading to cognitive impairment and the characteristic symptoms of Alzheimer’s disease.
While the exact mechanisms underlying the link between inflammation and Alzheimer’s disease are yet to be fully understood, ongoing research suggests that chronic inflammation may contribute to the accumulation of amyloid-beta plaques and neurofibrillary tangles, the hallmarks of the disease. Inflammatory responses triggered by various factors, such as infections or injuries, can disrupt the delicate balance of the brain and set off a cascade of events that promote neurodegeneration.
- One potential pathway through which inflammation affects Alzheimer’s disease is the activation of immune cells, such as microglia and astrocytes, in the brain. These cells play a crucial role in the innate immune response and are responsible for monitoring and maintaining the brain’s microenvironment. However, when activated chronically, they can produce excessive inflammatory molecules that contribute to neuronal damage.
- Inflammatory mediators, including cytokines and chemokines, have been found to be elevated in the brains of Alzheimer’s patients. These molecules can modulate the immune response and impact neuronal function, potentially exacerbating the disease progression. Researchers are investigating strategies to target and regulate these inflammatory mediators to mitigate the detrimental effects on brain health.
- Furthermore, recent studies have highlighted the involvement of the blood-brain barrier (BBB) in the inflammatory processes associated with Alzheimer’s disease. The BBB acts as a protective barrier, regulating the entry of substances into the brain. Disruption of the BBB integrity can allow the infiltration of immune cells and pro-inflammatory molecules, intensifying neuroinflammation and contributing to neurodegeneration.
Understanding the role of inflammation in Alzheimer’s disease is crucial for the development of effective therapeutic interventions. Targeting inflammatory pathways and modulating immune responses may offer potential avenues for preventing or slowing down the progression of this devastating neurodegenerative disorder.
Iron’s Influence on Inflammatory Processes
Exploring the association between iron and inflammatory processes sheds light on the intricate relationship between these two entities.
The impact of iron on inflammatory processes is a topic of growing interest and research in the field of Alzheimer’s disease. Various studies have highlighted the profound influence of iron on the intricate network of inflammatory pathways within the brain.
Iron’s involvement in inflammatory responses encompasses both its essential role as a cofactor in numerous metabolic processes and its potential to contribute to oxidative stress. These dual aspects contribute to the complex interplay between iron and inflammation that can significantly affect the progression of neurodegenerative disorders such as Alzheimer’s disease.
Activation of microglia, the resident immune cells in the brain, plays a vital role in the initiation and regulation of inflammatory processes. Perturbations in iron homeostasis have been shown to modulate microglial activation and subsequent release of pro-inflammatory cytokines, thereby setting off a cascade of immune responses that can impact neuronal health and function.
Moreover, iron’s ability to stimulate the generation of reactive oxygen species can further exacerbate inflammatory processes. These highly reactive molecules contribute to the oxidative damage observed in Alzheimer’s disease and are believed to promote the production of amyloid-beta plaques, a hallmark feature of this neurodegenerative disorder.
In summary, understanding the influence of iron on inflammatory processes provides valuable insights into the complex pathophysiology of Alzheimer’s disease. By unraveling the intricate mechanisms through which iron contributes to neuroinflammation, researchers can potentially identify novel therapeutic targets to mitigate the progression of this devastating disease.
Neuroinflammation as a Result of Iron Dysregulation
Neuroinflammation can arise due to the disruption in the balance of iron levels within the brain. Fluctuations in iron homeostasis can lead to an inflammatory response in the neurological system, involving the activation of immune cells and the release of pro-inflammatory molecules.
The dysregulation of iron within the brain can stimulate various immune cells, including microglia and astrocytes, which are responsible for maintaining the health and function of neurons. When iron levels become imbalanced, these immune cells can become overactivated and trigger a cascade of inflammatory processes.
- This neuroinflammatory response can result in significant damage to neurons and their connections, affecting cognitive abilities and contributing to the pathogenesis of neurodegenerative diseases.
- Iron dysregulation-induced neuroinflammation has been implicated in the progression of Alzheimer’s disease, albeit there is still much to uncover regarding the precise mechanisms involved.
- Studies have shown that high iron levels in the brain can lead to the accumulation of amyloid-beta plaques, a hallmark of Alzheimer’s disease.
- Additionally, iron dysregulation can promote oxidative stress and mitochondrial dysfunction, further exacerbating neuroinflammatory responses.
- Understanding the intricate relationship between iron dysregulation and neuroinflammation is crucial for developing therapeutic interventions that target these mechanisms to potentially prevent or attenuate the progression of neurodegenerative diseases.
Further research is needed to elucidate the specific pathways and molecular interactions involved in the development of neuroinflammation as a result of iron dysregulation. By gaining a deeper understanding of these processes, novel strategies can be devised to modulate iron homeostasis and alleviate the detrimental effects of neuroinflammation in Alzheimer’s disease and other related conditions.
Genetic Factors and Iron-Related Abnormalities
Exploring the intricate connection between genetic factors and iron-related abnormalities can shed light on the complex mechanisms underlying the development of Alzheimer’s disease. Understanding how specific genes and iron dysregulation contribute to this neurodegenerative disorder is vital for unraveling the enigma surrounding its pathology.
Multiple genes have been identified that play a role in iron metabolism and transport, and their variants have been associated with an increased risk of developing Alzheimer’s disease. These genes, often referred to as genetic susceptibility factors, influence the body’s ability to regulate iron levels effectively. Disruptions in iron homeostasis, such as increased iron accumulation or deficiencies, can lead to oxidative stress and contribute to the formation of amyloid plaques and neurofibrillary tangles, hallmark pathologies of Alzheimer’s disease.
Moreover, iron-related abnormalities extend beyond genetic factors, encompassing environmental influences as well. Excessive iron intake or exposure to environmental toxins can further disrupt iron homeostasis, exacerbating the risk of developing Alzheimer’s disease. Understanding the interplay between genetic predispositions and environmental factors offers valuable insights into personalized approaches for disease prevention and treatment.
An important aspect of genetic research in Alzheimer’s disease revolves around the identification of susceptibility genes that modulate iron metabolism pathways. By pinpointing these genetic factors, researchers can better comprehend the intricate interplay between iron dyshomeostasis and the development of Alzheimer’s disease. Furthermore, these findings can facilitate the development of novel therapeutic targets aimed at restoring iron balance and mitigating the progression of this debilitating condition.
- Research has demonstrated that variations in genes encoding proteins involved in iron transport and storage, including transferrin, ferritin, and the HFE gene, are associated with an increased risk of Alzheimer’s disease.
- Genetic mutations affecting the production or function of these iron-related proteins have been shown to disrupt iron homeostasis and contribute to neurodegeneration.
- Understanding the genetic underpinnings of iron-related abnormalities can pave the way for targeted interventions, such as gene therapies or iron chelation strategies, that aim to restore normal iron levels and mitigate neurotoxicity.