The Impact of Quantum Computing on the Future of Technology
Quantum Computing: Revolutionizing Technology and Unlocking New Frontiers
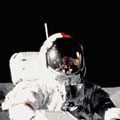
- Anthony Arphan
- 30 min read
In the intricate realm of cutting-edge technology, an extraordinary progression of innovation is reshaping our understanding of science and computation. This revolutionizing force, known as quantum computing, has emerged as a groundbreaking platform that transcends traditional computational boundaries. Through the manipulation of subatomic particles, this nascent field has unlocked the potential for exponential growth, revolutionizing not only the technological landscape but also our philosophical understanding of the universe.
Embarking on a Journey into Uncharted Territory
The enigmatic realm of quantum computing beckons adventurers eager to explore the transcendent possibilities it holds. By harnessing the elusive properties of matter at the atomic and subatomic levels, quantum computing offers a paradigm shift that propels us into the realm of exponential computational power, enabling us to solve complex problems at an unimaginable pace. As traditional computing principles reach their limits, this emerging field has set ablaze a new era of digital innovation and discovery.
Unleashing the Power of Quantum Prowess
Embracing the immense capabilities of quantum computing, scientists and researchers are on a quest to unravel the mysteries of the universe. With subatomic particles functioning as the building blocks of computation, the potential for accelerated artificial intelligence, optimized data analysis, and revolutionary encryption methods becomes tantalizingly within reach. By leveraging the principles of entanglement and superposition, quantum computing presents a gateway to unlocking the secrets hidden in large and complex datasets, revolutionizing simulations, and enabling breakthroughs in various scientific disciplines.
As this thrilling expedition into the quantum realm unfolds, we find ourselves standing at the precipice of an extraordinary revolution. Quantum computing is poised to redefine the limits of human capability, propelling us towards a future where unimaginable technological advancements merge seamlessly with our quest for knowledge and understanding. Brace yourselves, as the quantum revolution is upon us!
Understanding Quantum Computing
Delving into the realm of cutting-edge innovation, we explore the fascinating field of quantum computing. This revolutionary discipline is poised to transform the way we process information, unravelling the potential of quantum phenomena to revolutionize computing capabilities.
What makes quantum computing unique?
Unlike conventional computing, which relies on classical bits, quantum computing harnesses the power of quantum bits, or qubits, to transcend the limitations of binary code. By utilizing quantum superposition and entanglement, qubits can exist in multiple states simultaneously, offering unparalleled computational possibilities.
The quantum advantage:
Quantum computing’s potential lies in its ability to tackle complex problems with unprecedented efficiency. Harnessing the power of quantum parallelism, quantum algorithms hold the promise of exponentially faster computation for tasks such as optimization, simulation, and cryptography.
Understanding the challenges:
While the concept of quantum computing holds immense promise, its realization faces significant obstacles. Maintaining the fragile coherence of qubits, minimizing errors due to environmental disturbances, and scaling up to large-scale quantum systems pose formidable challenges that researchers are tirelessly working to overcome.
The practical implications:
As quantum computing advances, it is expected to revolutionize various industries. From enhancing drug discovery and optimizing logistics to revolutionizing financial modeling and cryptography, the impact of quantum computing is poised to reshape the technological landscape in profound ways.
In conclusion, gaining a foundational understanding of quantum computing is essential to appreciate its transformative potential and the paradigm shift it offers in the realms of computation. Embracing the principles of quantum mechanics, this emerging field holds the key to unlocking extraordinary computational power and paving the way for groundbreaking technological advancements.
The basics of quantum mechanics
In this section, we will explore the fundamental concepts and principles that form the foundation of quantum mechanics, an intriguing branch of physics that unveils the mysteries of the microscopic world. Through the lens of quantum mechanics, we venture into a realm where particles can exist in multiple states simultaneously, where uncertainty and probability reign, and where traditional rules of classical physics no longer hold true.
Quantum mechanics, often regarded as the backbone of quantum computing, is a formidable discipline that studies the behavior of matter and energy on an atomic and subatomic scale. Its core tenets challenge our intuition and defy the conventions of classical physics. Instead of relying on fixed states and determinism, quantum mechanics introduces the concept of wave-particle duality, where objects can exhibit both wave-like and particle-like characteristics.
Superposition is a crucial principle in quantum mechanics. It describes the ability of particles to adopt multiple states simultaneously, each with a certain probability. Unlike classical bits, which can only represent a definite 0 or 1, quantum bits or qubits can exist in a superposition of both states, allowing for a vast increase in computational possibilities.
Moreover, entanglement, another fascinating aspect of quantum mechanics, establishes a profound connection between particles, even when separated by vast distances. When particles become entangled, their properties become intrinsically linked, regardless of the physical distance between them. This phenomenon, famously referred to by Einstein as “spooky action at a distance,” has tremendous implications for communication, encryption, and the future of computing.
Understanding the basics of quantum mechanics sets the stage for comprehending the inner workings of quantum computing and the revolutionary potential it holds. By embracing the counterintuitive and often mind-boggling principles of quantum mechanics, scientists are paving the way towards an unprecedented era of technological advancement and discovery.
The difference between classical and quantum computing
In the realm of computing, there exists two distinct paradigms that shape the landscape of technological advancements. These paradigms are known as classical computing and quantum computing. While both are aimed at solving complex problems and performing calculations, they differ in their fundamental principles and capabilities.
Classical computing, as we know it today, relies on the binary system of digits, 0 and 1, to represent information. It follows a deterministic approach, where the outcome of a computation can be predicted with certainty based on the initial conditions and set of instructions given. Classical computers process data using bits, which can exist in only one state at a time, either 0 or 1. These bits are manipulated through logical operations, allowing for tasks such as arithmetic calculations, data storage, and logical decision-making.
Quantum computing, on the other hand, harnesses the principles of quantum mechanics, opening up a whole new realm of possibilities. Instead of using bits, quantum computers utilize quantum bits, or qubits, which can exist in a superposition of states. This means that a qubit can simultaneously represent both 0 and 1, exponentially increasing the computational power. Quantum computers also leverage an intriguing property called entanglement, where qubits can become linked in such a way that the state of one qubit affects the state of another, even if they are physically separated.
The potential of quantum computing lies in its ability to perform calculations at an astonishing rate, tackling complex problems that are practically infeasible for classical computers. This includes tasks such as simulating the behavior of molecules, optimizing complex systems, and breaking cryptographic algorithms, to name a few examples. As quantum computing continues to advance, it has the potential to revolutionize various industries, including pharmaceuticals, finance, and artificial intelligence, by providing faster and more efficient solutions to intricate computational problems.
In conclusion, the distinction between classical and quantum computing lies in their underlying principles and capabilities. While classical computing relies on the binary system and deterministic operations, quantum computing harnesses the power of qubits and quantum mechanics, enabling the exploration of an exponentially larger solution space. As researchers continue to make breakthroughs and overcome technical challenges, the future of quantum computing holds great promise for the advancement of technology.
The potential of quantum computing
Exploring the vast possibilities that lie within the realm of quantum computing opens up unprecedented opportunities for innovation and advancement in various fields. Quantum computing, with its unique approach to computation, has the potential to revolutionize diverse industries, transcend current limitations, and unlock new frontiers in scientific and technological achievements.
By harnessing the principles of quantum mechanics, quantum computing offers the capability to process and manipulate vast amounts of information simultaneously through the use of quantum bits, or qubits. These qubits, unlike traditional bits, can exist in multiple states simultaneously, allowing for exponentially faster computations and the ability to analyze complex problems that are currently beyond the reach of classical computers.
One of the most promising applications of quantum computing lies in the field of cryptography. Quantum computers possess the ability to break traditional cryptographic algorithms, rendering current security measures vulnerable. However, they also provide the potential to develop unbreakable encryption methods, ensuring secure communication and data protection on an unprecedented scale.
Additionally, quantum computing holds the promise of transforming optimization problems that plague numerous industries. From supply chain management to financial modeling, the ability of quantum computers to rapidly process vast amounts of data and solve complex optimization challenges can result in significant improvements in efficiency, cost savings, and strategic decision-making.
Moreover, quantum computing’s potential impact stretches beyond traditional computing domains. Its ability to simulate quantum systems with high accuracy opens up new avenues for scientific research, allowing scientists to better understand quantum phenomena, simulate chemical reactions, and design new materials with extraordinary properties.
As quantum computing technology continues to advance, its potential impact on society and the world as a whole cannot be understated. The transformative power of quantum computing has the potential to reshape industries, revolutionize scientific discoveries, and drive innovation to unimaginable heights.
Quantum Computing Applications
Advancements in the emerging field of quantum computing have opened up a wide range of potential applications across various industries. This section explores the practical applications that harness the unique properties of quantum mechanics to address complex problems and revolutionize traditional computing paradigms.
Application | Description |
---|---|
Optimization | Quantum computing algorithms have the potential to significantly improve optimization processes, enabling more efficient resource allocation, route planning, scheduling, and supply chain management. |
Drug Discovery | By leveraging quantum computing’s ability to analyze vast amounts of data and complex molecular interactions, researchers can accelerate the development of new drugs and explore personalized medicine options. |
Cryptography | Quantum computing offers both opportunities and challenges for cryptography. Quantum-resistant encryption algorithms can be developed to secure communications and data exchanges, while quantum computers may also be used to break existing cryptographic systems. |
Machine Learning | Quantum machine learning algorithms have the potential to enhance pattern recognition, data analysis, and predictive modeling, enabling more advanced artificial intelligence systems across various domains, including finance, healthcare, and transportation. |
Financial Modeling | Quantum computing can revolutionize financial modeling by enabling more accurate risk assessment, portfolio optimization, asset pricing, and algorithmic trading strategies, ultimately enhancing decision-making and performance in the financial industry. |
Climate Modeling | Quantum computing can enhance climate modeling capabilities by simulating complex climate systems with higher accuracy and speed. This can assist in predicting and addressing climate change-related challenges, such as extreme weather events and rising sea levels. |
These are just a few examples showcasing the potential impact of quantum computing applications. As advancements continue to be made in this field, the possibilities for leveraging quantum computing to solve complex problems and drive innovation are limitless.
Quantum Cryptography and Secure Communication
In the realm of cutting-edge technological advancements, the field of quantum cryptography emerges as a paramount breakthrough. With the ever-growing need for secure communication in today’s interconnected world, quantum cryptographic solutions are paving the way for a new era of impervious data protection. This section delves into the profound implications and potential of utilizing the principles of quantum mechanics to establish unbreakable encryption keys and safeguard information.
At its core, quantum cryptography leverages the fundamental principles of quantum mechanics, such as superposition and entanglement, to ensure secure communication. Unlike classical cryptography, which relies on the computational difficulty of factorization or discrete logarithm problems, quantum cryptography exploits the inherent properties of quantum particles to guarantee the confidentiality and integrity of transmitted data.
One of the cornerstones of quantum cryptography is the concept of quantum key distribution (QKD). QKD enables the generation of encryption keys that are secure against any potential computational attack. By utilizing the principles of quantum mechanics, QKD allows two parties to establish a shared encryption key without the need to transmit it over a potentially compromised channel.
Another compelling aspect of quantum cryptography is its ability to detect eavesdropping attempts. Utilizing the phenomenon called the observer effect, any interception or measurement of quantum information inherently alters its state, thus making it detectable. This provides an additional layer of security by signaling the presence of an unauthorized third party attempting to gain access to the encrypted information.
The potential impact of quantum cryptography extends beyond traditional communication channels. As industries and governments increasingly rely on the integrity of digital transactions and the protection of sensitive information, quantum cryptography emerges as an indispensable solution. Applications range from secure data transmission in financial systems to safeguarding classified government communications, ensuring that the essential pillars of our modern society remain protected.
In conclusion, the realm of quantum cryptography offers revolutionary advancements in secure communication. By harnessing the principles of quantum mechanics, it provides unprecedented levels of data protection, unbreakable encryption, and the ability to detect any unauthorized access. As technology continues to advance, the integration of quantum cryptography into our digital infrastructure will play a crucial role in ensuring the safety and confidentiality of our information in the years to come.
Simulating complex chemical reactions and drugs
In the ever-evolving landscape of scientific advancement, computational tools have emerged as valuable assets in simulating complex chemical reactions and studying the intricate behavior of drugs. By harnessing the power of cutting-edge technologies, scientists can now explore the intricate interaction of particles, analyze reaction pathways, and predict the behavior of chemical compounds.
This field of research, often referred to as computational chemistry, has become a fascinating realm where scientists leverage the capabilities of quantum computing to unlock new understanding and possibilities. Through the collaboration of researchers, chemists, and computer scientists, computational chemistry is uncovering valuable insights into the molecular world that were previously unimaginable.
Simulating complex chemical reactions and drugs involves the use of sophisticated algorithms and software that utilize quantum principles. These simulations enable scientists to investigate the potential configurations and properties of various chemical compounds, allowing for rapid and cost-effective work in the discovery and design of novel drugs.
One of the main advantages of quantum computing in these simulations is its ability to handle the vast complexity involved in studying chemical reactions. By exploiting the principles of quantum mechanics, researchers can simulate intricate molecular systems, identify potential drug targets, and even optimize drug formulations. This computational power complements experimental approaches, accelerating the drug discovery process and offering a more comprehensive understanding of chemical processes.
Beyond drug discovery, simulating complex chemical reactions also holds promise in various fields such as materials science, energy production, and environmental sustainability. The ability to accurately model chemical reactions and predict their outcomes opens up avenues for developing new materials, improving energy storage systems, and designing more efficient catalysts.
In conclusion, the intersection of quantum computing and computational chemistry has propelled the simulation of complex chemical reactions and drugs into new realms. The immense computational power and predictive capabilities offered by quantum computing enable scientists to unlock novel insights and accelerate advancements across various scientific disciplines. As technology continues to evolve, the potential for simulating and understanding complex chemical systems becomes even greater, paving the way for groundbreaking discoveries and innovations.
Optimizing logistical problems and supply chains
In an era of rapid technological advancements, optimizing logistical problems and supply chains has become crucial for businesses. With the emergence of quantum computing, new opportunities are arising to revolutionize the way we manage and streamline these essential processes.
By harnessing the power of quantum computing, organizations can tackle complex logistical challenges with greater efficiency and precision. Traditional computing methods often struggle to handle the immense amounts of data involved in supply chain management. Quantum computing, on the other hand, offers the potential for exponential computational power, enabling faster and more accurate analysis of vast datasets.
One area where quantum computing can offer significant improvements is in route optimization. Supply chains involve intricate networks of transportation routes, and optimizing these routes is key to minimizing costs and maximizing efficiency. Quantum algorithms can evaluate countless variables simultaneously, allowing for the identification of the most optimal routes in real-time. This can lead to substantial time and cost savings for businesses.
Furthermore, quantum computing can also help optimize inventory management. Supply chains often deal with numerous variables, including demand fluctuations, lead times, and production capacities. Quantum algorithms can analyze these variables and predict optimal inventory levels to ensure smooth operations. By reducing excess inventory and preventing shortages, businesses can avoid unnecessary costs and maintain customer satisfaction.
Another potential application of quantum computing in supply chains is in demand forecasting. Accurately predicting customer demand is essential for avoiding overstocking or understocking of products. Through analyzing historical data and incorporating external factors, quantum algorithms can generate more accurate demand forecasts. This can enable businesses to make better-informed decisions regarding production planning and inventory management, leading to improved customer service and increased profitability.
In conclusion, as quantum computing continues its advancements, optimizing logistical problems and supply chains presents an exciting opportunity for businesses. The ability to process vast amounts of data and identify optimal solutions in real-time can revolutionize the way organizations manage their supply chains. By leveraging the power of quantum algorithms, businesses can achieve greater efficiency, reduced costs, and enhanced customer satisfaction in the ever-growing global marketplace.
Quantum Computing Challenges
Exploring the potential of the quantum realm and harnessing its power to revolutionize technology is an exciting endeavor, but it is not without its challenges. As researchers and scientists delve deeper into the world of quantum computing, they encounter numerous obstacles that need to be overcome in order to fully harness the potential of this groundbreaking technology.
One of the major challenges in the field of quantum computing is the issue of qubit stability. Unlike classical bits, which can represent a binary value of either 0 or 1, qubits can exist in a superposition of states, representing both 0 and 1 simultaneously. However, due to their delicate nature, qubits are highly susceptible to disturbance from external factors such as temperature fluctuations and electromagnetic interference. Maintaining the stability of qubits is crucial for performing complex computations and preventing errors in quantum algorithms.
Another challenge is the issue of qubit connectivity. In classical computing, bits can communicate with each other seamlessly, allowing for efficient data processing. In quantum computing, qubits need to be interconnected in order to perform complex calculations. However, creating and maintaining these connections poses a significant technical hurdle. Scaling up the number of qubits and ensuring their connectivity is a complex task that requires innovative solutions.
Furthermore, quantum decoherence presents a significant challenge in quantum computing. Decoherence refers to the loss of quantum states due to interaction with the environment. As qubits interact with their surroundings, their delicate quantum states can quickly degrade, leading to errors in calculations. Researchers are developing techniques to minimize the impact of decoherence and extend the coherence times of qubits, but it is still an ongoing challenge that needs to be addressed for practical quantum computing.
Additionally, quantum error correction is a vital challenge in the field. Quantum systems are highly sensitive to errors, and even tiny perturbations can result in catastrophic failures of quantum computations. Developing efficient error correction techniques to detect and correct errors is essential for reliable and accurate quantum computing.
Quantum Computing Challenges |
---|
- Qubit Stability |
- Qubit Connectivity |
- Quantum Decoherence |
- Quantum Error Correction |
Overcoming Quantum Decoherence
In the realm of quantum computing, an inherent challenge that researchers and developers face is the phenomenon known as quantum decoherence. This obstacle poses a significant barrier to the stability and reliability of quantum systems, hindering their effectiveness in practical applications. However, scientists are diligently working towards finding solutions to overcome this hurdle and unlock the full potential of quantum computing.
Quantum decoherence refers to the phenomenon wherein a quantum system loses its delicate state of superposition and entanglement due to interactions with its surrounding environment. This interaction leads to the breakdown of the quantum system’s coherent behavior, resulting in the loss of valuable quantum information. Consequently, this decoherence greatly limits the lifespan of quantum states, inhibiting the stability and accuracy required for practical quantum computations.
To address the challenge posed by quantum decoherence, researchers are exploring various strategies and techniques. One approach involves implementing quantum error correction codes, which employ redundancy to protect against errors induced by decoherence. By encoding quantum information in a way that enables error detection and correction, these codes offer a potential solution for combating the detrimental effects of decoherence.
Another avenue of research focuses on developing error-resistant qubits, the fundamental building blocks of quantum computers. Scientists are investigating methods to engineer stable qubits that are less susceptible to external disturbances and intrinsic decoherence. Novel materials and fabrication techniques are being explored to create qubits with longer coherence times, thereby mitigating the impact of decoherence on quantum computations.
In addition to these hardware-based approaches, software solutions are also being developed to overcome quantum decoherence. Quantum algorithms are being designed to take into account potential errors induced by decoherence, utilizing error-tolerant techniques to achieve accurate results despite the presence of noise and disruption. These algorithmic advancements aim to minimize the impact of decoherence on quantum computations, enhancing their resilience and practicality.
Overcoming quantum decoherence is a crucial step towards harnessing the true power of quantum computing. By addressing this challenge, researchers and developers pave the way for the realization of applications with profound implications in fields such as cryptography, optimization, drug discovery, and more. As the quest to conquer decoherence continues, the future of quantum computing holds great promise in revolutionizing the technological landscape.
Developing error correction techniques
In the realm of advancing technology, there is a growing need to address and rectify inaccuracies that arise in quantum computing systems. Error correction techniques are a vital aspect of this process, as they aim to mitigate the effects of errors that inevitably occur during the computation process.
The development of error correction techniques in quantum computing involves the exploration and implementation of innovative approaches to overcome the inherent challenges posed by quantum systems. These techniques aim to enhance the reliability and accuracy of quantum computations, enabling quantum computers to provide more consistent and dependable results.
One prominent method in error correction is the utilization of quantum error correction codes. These codes enable the detection and correction of errors that occur in quantum states, similar to the error correction codes used in classical computing. Quantum error correction codes consist of encoding schemes that distribute the information across multiple qubits, providing redundancy that facilitates error detection and correction.
Another avenue of research in developing error correction techniques involves error-detecting algorithms and error-detecting codes. These algorithms and codes are designed to identify and address errors in quantum computations, leveraging mathematical principles and statistical methods to detect when errors occur and take appropriate corrective action.
Furthermore, the development of fault-tolerant quantum computing systems is a key aspect of error correction techniques. These systems are designed to both detect and mitigate errors in real-time, allowing for the continued execution of quantum computations even in the presence of errors. Fault-tolerant systems incorporate redundancy and redundancy-checking mechanisms to ensure the integrity and accuracy of quantum computations.
Error Correction Techniques in Quantum Computing |
---|
- Quantum error correction codes |
- Error-detecting algorithms and codes |
- Fault-tolerant quantum computing systems |
Overall, the development of error correction techniques in quantum computing is imperative for the advancement and practical implementation of this cutting-edge technology. By addressing and mitigating errors, these techniques pave the way for more reliable and accurate quantum computations, which in turn opens up new possibilities and opportunities in various fields, such as optimization, cryptography, and drug discovery.
Scaling up quantum systems
As we look into the future of advancing technology, one area that continues to garner significant attention is the scaling up of quantum systems. Quantum computing, with its potential to revolutionize various industries and solve complex problems more efficiently, is paving the way for a new era of computation.
When it comes to scaling up quantum systems, the focus is on expanding the number of qubits and improving their reliability and stability. Qubits, the fundamental building blocks of quantum computers, harness the principles of quantum mechanics to store and process information. The ability to scale up these qubits is essential for achieving larger and more powerful quantum systems.
However, scaling up quantum systems is not without its challenges. One significant obstacle is dealing with the inherent fragility of qubits and their susceptibility to noise and interference from the external environment. Scientists and researchers are working tirelessly to develop techniques and technologies that can mitigate these challenges and improve the robustness and stability of qubits at scale.
Another aspect of scaling up quantum systems involves developing more efficient methods for controlling and manipulating qubits. This includes advancements in error correction techniques, as well as finding ways to reduce the error rates during quantum operations. These improvements are necessary to ensure the accuracy and reliability of the computations carried out by quantum systems as they grow in size.
Furthermore, the physical infrastructure required to support scaled-up quantum systems needs to be considered. This includes designing and building specialized quantum computing hardware, as well as establishing the necessary infrastructure for supporting the storage and transmission of quantum information.
Current Quantum Computing Research
In the rapidly evolving field of quantum information science, researchers are actively exploring various aspects of quantum computing. This section aims to provide an overview of the ongoing research initiatives in this exciting realm.
Scientists and engineers are delving into the exploration of quantum algorithms, seeking to develop new computational techniques that can solve complex problems more efficiently than classical computers. These algorithms harness the principles of quantum mechanics, enabling the potential for exponential speedup in solving certain computational tasks.
Another area of current research involves the advancement of quantum hardware. Scientists are actively designing and fabricating quantum processors, aiming to enhance their stability, coherence, and scalability. These quantum hardware systems utilize quantum bits, or qubits, as the fundamental units of information processing, and research is focused on improving their precision and reducing errors.
Furthermore, researchers are investigating the development of quantum error correction codes to mitigate the effects of environmental noise and errors that naturally occur in quantum systems. This is crucial for ensuring the reliability and scalability of quantum computers, as quantum information is highly susceptible to decoherence.
Additionally, efforts are being made to cultivate quantum simulators, which are specialized quantum systems that can accurately model and simulate the behavior of other quantum systems. These simulators enable researchers to explore and understand complex quantum phenomena, paving the way for advancements in fields such as materials science and chemistry.
Quantum communication and cryptography are also areas of active research, aiming to develop secure and efficient methods for transmitting and protecting quantum information. Quantum key distribution protocols are being explored to enable secure communication channels that are resistant to hacking attempts.
Lastly, researchers are investigating the potential applications of quantum computing in various fields, including optimization, machine learning, finance, and drug discovery. These explorations aim to harness the power of quantum computing to revolutionize traditional approaches and drive innovation in diverse domains.
Research Areas | Description |
---|---|
Quantum algorithms | Exploration of computational techniques leveraging principles of quantum mechanics |
Quantum hardware | Advancement in the design and fabrication of quantum processors and qubits |
Quantum error correction | Development of codes to mitigate errors and decoherence in quantum systems |
Quantum simulators | Specialized systems for accurately modeling and simulating quantum phenomena |
Quantum communication and cryptography | Secure methods for transmitting and protecting quantum information |
Applications of quantum computing | Exploring potential use cases in optimization, machine learning, finance, and drug discovery |
Quantum Supremacy and Its Significance
Quantum supremacy, an authoritative breakthrough in the field of quantum computing, represents a pivotal moment in technological advancement and has far-reaching implications for various industries and scientific disciplines. This section explores the meaning and implications of quantum supremacy, discussing its potential to revolutionize computing capabilities, enhance data analysis, and solve complex problems that classical computers find insurmountable.
Quantum supremacy signifies a state where quantum computers outperform classical ones, demonstrating computational power that is beyond the reach of even the most advanced traditional machines. This achievement stems from the unique properties of quantum systems, such as superposition and entanglement, allowing for exponential computational speed and parallel processing.
The implications of quantum supremacy extend to numerous fields, including cryptography, optimization, chemistry, and materials science. In the realm of cryptography, quantum computers could potentially render conventional encryption methods obsolete and require the development of quantum-resistant algorithms to ensure data security. Additionally, quantum supremacy opens up new avenues in optimization, enabling faster and more efficient solutions for complex logistical challenges and optimization problems.
- It promises advancements in drug discovery and materials science by accelerating simulations and modeling, leading to the development of more efficient medications and innovative materials.
- Quantum supremacy has the potential to revolutionize financial systems by enabling faster and more accurate risk analysis, portfolio optimization, and fraud detection.
- The impact of quantum supremacy on artificial intelligence and machine learning is also significant. Quantum algorithms have the potential to enhance pattern recognition, optimization of neural networks, and complex data analysis, opening doors to new breakthroughs in AI applications.
However, achieving and harnessing quantum supremacy is not without challenges. Overcoming technical hurdles, such as quantum decoherence, error correction, and scalability, is crucial for realizing the full potential of quantum computing.
In conclusion, quantum supremacy represents a paradigm shift in the capabilities of computing systems, offering immense possibilities for various industries and scientific endeavors. Its implications extend to fields as diverse as cryptography, optimization, drug discovery, finance, and artificial intelligence. While challenges remain, the pursuit of quantum supremacy promises to reshape our understanding of computational power and forge a new era of technological innovation.
Major breakthroughs in quantum algorithms
In the realm of quantum computing, significant advancements have been achieved through the exploration and development of revolutionary algorithms. These breakthroughs have propelled the field forward, enabling quantum computers to solve complex problems with unprecedented speed and efficiency.
One notable breakthrough lies in the development of quantum search algorithms. Unlike classical search algorithms, which require time proportional to the size of the dataset being searched, quantum search algorithms such as Grover’s algorithm offer the potential for exponential speedup. By leveraging quantum properties such as superposition and interference, these algorithms efficiently locate desired solutions from a vast number of possibilities.
Another exciting breakthrough is the invention of quantum factoring algorithms. Factoring large numbers is a crucial component of many encryption systems, and classical algorithms have traditionally struggled to factorize numbers of significant magnitude. However, with the creation of Shor’s algorithm, quantum computers can factorize large numbers in polynomial time. This breakthrough has the potential to render many encryption methods obsolete, as quantum computers will possess the capability to decipher encrypted data at an unprecedented pace.
The development of quantum simulation algorithms is yet another breakthrough that holds immense promise. Quantum simulators can accurately model complex quantum systems that are beyond the reach of classical computers. Applications of quantum simulation algorithms span a wide range of fields, including chemistry, physics, and materials science. With these algorithms, scientists can explore and study quantum phenomena, leading to groundbreaking discoveries and advancements in various scientific disciplines.
- Quantum search algorithms such as Grover’s algorithm offer exponential speedup in finding desired solutions
- Shor’s algorithm enables quantum computers to factorize large numbers efficiently, threatening traditional encryption methods
- Quantum simulation algorithms allow accurate modeling of complex quantum systems, leading to advancements across multiple scientific fields
These major breakthroughs in quantum algorithms represent significant strides towards harnessing the full potential of quantum computing. As further research and development continue, the application of these groundbreaking algorithms will undoubtedly shape the future of technology and revolutionize various industries in unimaginable ways.
Quantum Computing in the Cloud
Exploring the potential of quantum computing and its impact on the future of technology involves delving into various aspects, one of which is quantum computing in the cloud. This emerging field brings together the power of quantum computing and the convenience of cloud computing, creating new possibilities for researchers, businesses, and individuals alike.
The integration of quantum computing into the cloud allows for improved accessibility and scalability. By harnessing the power of quantum computers through cloud-based platforms, users can access quantum resources remotely, reducing the need for expensive infrastructure on their own premises. This opens up avenues for collaboration and innovation, as researchers from different parts of the world can connect and work together on quantum projects.
Additionally, quantum computing in the cloud offers enhanced security and privacy. Quantum encryption algorithms can provide stronger protection to sensitive information, making it significantly more challenging for unauthorized individuals to intercept or decrypt data. This feature has the potential to transform industries that rely heavily on secure communication, such as finance, government, and healthcare.
Furthermore, the cloud-based nature of quantum computing allows for improved scalability and flexibility. As quantum technology advances, more and more quantum devices can be added to the cloud infrastructure, providing users with access to increasingly powerful quantum computing capabilities. This scalability opens up possibilities for solving complex problems in fields such as optimization, drug discovery, and weather forecasting.
- Increased Accessibility: Quantum resources can be accessed remotely, removing the need for expensive infrastructure.
- Enhanced Security: Quantum encryption algorithms provide stronger protection to sensitive information.
- Improved Scalability: The cloud-based environment allows for the addition of more powerful quantum devices as technology progresses.
In conclusion, quantum computing in the cloud is revolutionizing the landscape of technology by providing increased accessibility, enhanced security, and improved scalability. As the field continues to advance, more applications and advancements are expected, leading to a future where quantum computing becomes an integral part of our everyday lives.
Quantum Computing and Artificial Intelligence
The intersection of quantum computing and artificial intelligence (AI) holds immense potential for the future of technology. By harnessing the power of quantum mechanics, quantum computing has the ability to revolutionize AI by exponentially increasing computational speed and capacity. This powerful combination has the potential to transform industries, solve complex problems, and push the boundaries of what is possible.
Quantum computing takes advantage of the principles of quantum mechanics, which allows for the creation of quantum bits or qubits. Unlike classical bits, which can only represent either a 0 or a 1, qubits can exist in a superposition of both states simultaneously. This unique property allows quantum computers to process and manipulate vast amounts of information simultaneously, leading to unprecedented computational power.
This quantum advantage has the potential to greatly enhance various aspects of artificial intelligence. Machine learning algorithms, which form the backbone of AI, require massive computational capabilities to process and analyze large datasets. With quantum computing, these algorithms could be executed faster and more efficiently, accelerating the exploration and discovery of patterns and insights.
Additionally, the field of optimization, which seeks to find the best possible solutions to complex problems, can greatly benefit from the power of quantum computing. AI algorithms could leverage quantum optimization techniques to find optimal solutions in industries such as finance, logistics, and resource allocation. This could lead to significant improvements in efficiency, cost savings, and overall performance.
Furthermore, quantum computing could enable the development of more sophisticated AI models that can better understand and simulate complex systems. Quantum AI models could have the capability to analyze and simulate molecular structures, weather patterns, or even the behavior of financial markets with unprecedented accuracy. This could open up new avenues for scientific research, drug discovery, and risk management.
In conclusion, the convergence of quantum computing and artificial intelligence has the potential to reshape the future of technology. By harnessing the power of quantum mechanics, AI can reach new levels of computational speed and capacity, enabling advancements and breakthroughs in various industries. The transformational impact of this combination is not limited to a single field, but has the potential to revolutionize multiple aspects of our lives.
Enhancing machine learning algorithms with quantum computing
In this section, we explore the potential of quantum computing to revolutionize machine learning algorithms. By leveraging the unique properties of quantum systems, such as superposition and entanglement, researchers are discovering exciting possibilities for enhancing the capabilities of traditional machine learning techniques.
Quantum computing offers the promise of exponentially faster processing power, enabling the analysis and manipulation of vast amounts of data more efficiently than classical computers. This potential breakthrough has caught the attention of the machine learning community, as it opens up new avenues for tackling complex problems and improving predictive models.
The synergy between quantum computing and machine learning lies in their shared goal of extracting meaningful insights from data. Quantum algorithms can provide novel approaches to optimization and pattern recognition, allowing for more accurate predictions and faster decision-making processes.
One key advantage of quantum machine learning algorithms is their ability to handle high-dimensional data more efficiently. Traditional machine learning algorithms often struggle with the so-called “curse of dimensionality”, where the number of features in a dataset exceeds the available computational resources. By harnessing quantum computing techniques, we can potentially overcome this limitation and unlock new possibilities for analyzing complex datasets.
Furthermore, quantum machine learning algorithms have the potential to uncover hidden patterns and correlations in data that might not be easily discernible using classical approaches. This can lead to breakthroughs in areas such as image and speech recognition, drug discovery, and optimization problems.
In conclusion, the integration of quantum computing into machine learning holds great promise for advancing the field and pushing the boundaries of what is possible. By combining the power of quantum mechanics with the principles of artificial intelligence, we can unlock new frontiers of knowledge and revolutionize the way we solve complex problems.