Enhancing Structural Integrity - Exploring Methods and Alloys to Strengthen Iron
Enhancing Structural Integrity: Exploring Methods and Alloys to Strengthen Iron - Discover innovative techniques and alloys for fortifying iron and improving its structural integrity.
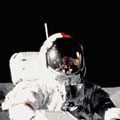
- Anthony Arphan
- 19 min read
In the realm of metallurgy, where strength and durability reign supreme, lies an ever-evolving quest to fortify iron and forge a more robust alloy. This pursuit unveils a fascinating world of innovation and exploration, where the boundaries of conventional techniques are continuously pushed. The intrinsic desire to enhance structural integrity fuels scientists, engineers, and artisans alike, leading them to unravel novel methods and ingenious alloys that epitomize the epitome of strength.
Within this intriguing domain, the art of crafting iron into a stalwart material emerges as a sublime fusion of science and creativity. The ardent endeavor encompasses a myriad of techniques borrowing from the realms of heat treatment, surface modifications, and alloy composition. Each method seeks to imbue iron with Herculean strength while preserving its inherent beauty and resilience.
Guided by the principles of strength and longevity, practitioners of this discipline employ a range of invigorating techniques en route to fortifying iron’s structural fortitude. Through precise manipulation of heat and cooling cycles, the molecular structure of iron is altered, imbuing it with exceptional hardness and toughness. Simultaneously, the deliberate modification of its surface properties enhances its resistance to corrosion and wear, ensuring longevity in the face of harsh environmental conditions.
Indeed, the fabrication of enhanced iron alloys serves as a veritable playground for those who seek to redefine the boundaries of strength. The ingenious combination of different metallic elements establishes alloys that surpass the limitations of pure iron, granting them enhanced mechanical properties. The incorporation of elements such as carbon, chromium, and nickel, among others, infuses iron with extraordinary strength, ductility, and resistance to deformation. This amalgamation of elements hails as a testament to human ingenuity, where the mere fusion of materials gifts iron with unprecedented levels of robustness.
Annealing Process
The Annealing Process section focuses on a fundamental technique that enhances the strength and durability of metal, specifically iron. This section delves into the concept behind the process without delving into specific definitions, providing readers with a comprehensive understanding of its significance in the context of metal engineering.
Annealing, a critical step in metal fabrication, is a heat treatment process that transforms the molecular structure of iron to increase its strength, hardness, and resistance to deformation. It involves heating the metal to a specific temperature and holding it at that temperature for a determined period, followed by controlled cooling. Through this controlled heating and cooling process, the metal undergoes internal changes that enhance its physical properties.
One of the main purposes of the annealing process is to relieve internal stresses within the metal. During the manufacturing process or due to external factors, such as welding, iron can accumulate stress and lose its strength. The annealing process allows for the redistribution of these internal stresses, resulting in a more uniform structure and improved mechanical properties.
Moreover, annealing facilitates grain growth and refinement, which can significantly impact the strength of iron. By heating the metal to the proper temperature range, smaller grains merge, forming larger, more closely packed grains. This refined microstructure provides enhanced resistance to deformation, as the larger grains offer a barrier against crack propagation and dislocation movement.
The annealing process also promotes the elimination of impurities and defects that may exist in the iron. By subjecting the metal to high temperatures, these impurities are driven out, leading to a purer and more homogeneous material. This purification process further contributes to the improved strength and integrity of the iron.
- Relieves internal stresses
- Facilitates grain growth and refinement
- Eliminates impurities and defects
In conclusion, the annealing process plays a crucial role in enhancing the strength and integrity of iron. By understanding this technique and its underlying principles, engineers and materials scientists can optimize the metal’s performance in various structural applications.
Heat Treatment
Enhancing the properties of metallic materials can be achieved through a process known as heat treatment. This method utilizes controlled heating and cooling procedures to modify the structure and properties of alloys in order to improve their performance in various applications.
During heat treatment, the material is subjected to carefully controlled temperature changes, often combined with specific cooling processes. These alterations induce changes in the microstructure of the alloy, resulting in enhanced mechanical properties such as strength, hardness, and resistance to deformation.
The specific heat treatment techniques employed depend on the desired outcome and the composition of the alloy. One common method is known as annealing, which involves heating the material to a specific temperature and then slowly cooling it to room temperature. This process relieves internal stresses and homogenizes the alloy’s microstructure, resulting in improved ductility and toughness.
Another heat treatment technique is quenching, where the alloy is rapidly cooled from a high temperature. This rapid cooling prevents the alloy from undergoing a full phase transformation, resulting in the formation of a harder microstructure with increased strength and hardness.
Tempering is yet another heat treatment process that involves reheating the quenched alloy to a specific temperature and then allowing it to cool slowly. This controlled cooling reduces the brittleness that can occur from quenching and provides a balance between hardness and toughness.
Overall, heat treatment techniques provide a means to optimize the mechanical properties of alloys, allowing for tailored strength, hardness, and other desirable characteristics. By carefully controlling the heating and cooling processes, engineers and metallurgists can impart enhanced structural integrity to iron and other alloys for a wide range of applications.
Reduction of Internal Stresses
In this section, we will delve into the methods and strategies employed to alleviate the internal stresses experienced within the material, aiming to enhance its overall structural integrity. Our focus lies on mitigating the tensions that occur within the substance, without relying solely on the conventional techniques or altering the composition of iron alloys. By employing innovative approaches, we aim to maintain the strength and durability of the material while minimizing the potential for internal stress-induced failures.
One of the key techniques employed to reduce internal stresses is through the process of stress relieving. This method entails subjecting the material to controlled heating and cooling cycles, strategically implemented to minimize the residual stresses within the iron structure. By carefully managing the temperature and duration of the treatment, we are able to relieve the accumulated stresses and achieve a more stable material with improved mechanical properties.
Another approach to reducing internal stresses involves the implementation of shot peening. This process consists of bombarding the surface of the material with small, spherical particles, such as steel shots or glass beads. The impact of these particles induces compressive stresses on the surface, which counteract the tensile stresses experienced within the material. Through shot peening, we can effectively enhance the fatigue strength and resistance to crack propagation, ultimately reducing the likelihood of internal stress-related failures.
Furthermore, the use of residual stress measurement techniques plays a crucial role in identifying any areas of high stress concentration within the material. By employing advanced non-destructive testing methods, such as X-ray diffraction or neutron diffraction, we can accurately map the distribution of internal stresses. This enables us to detect potential weak points and apply targeted stress relief techniques to ensure the overall integrity of the iron structure.
- Utilization of stress relieving techniques
- Implementation of shot peening
- Utilizing residual stress measurement techniques
By employing these innovative methods, it is possible to reduce the internal stresses within iron alloys, thereby enhancing their structural integrity. These strategies offer a promising avenue for the improvement of iron materials without compromising their inherent strength and durability.
Quenching and Tempering
In this section, we will delve into the process of quenching and tempering, which play a crucial role in enhancing the strength and durability of iron. These techniques involve carefully manipulating the cooling and heating processes of iron, resulting in a material that exhibits improved mechanical properties.
Quenching, also known as rapid cooling, is a method employed to harden iron by subjecting it to a rapid temperature change. By cooling the heated iron at a precise rate, we can lock in the desired crystalline structure, which contributes to enhanced strength. Quenching can be achieved using various mediums such as water, oil, or air, each offering different cooling rates and resulting in distinct material properties.
Tempering, on the other hand, involves reheating the quenched iron to a specific temperature and then cooling it slowly. This controlled process eliminates the brittleness that may have been introduced during quenching and brings the material to its optimal hardness and toughness. By carefully manipulating the tempering temperature, we can achieve a balance between the desired mechanical properties and the structural integrity of the iron.
The quenching and tempering process enables us to tailor the strength and hardness of iron to meet the requirements of various applications. Through careful selection of the quenching medium and tempering temperature, we can effectively control the microstructure and properties of the iron, improving its overall performance in structural applications.
Advantages | Disadvantages |
---|---|
- Enhanced strength | - Potential for distortion |
- Improved hardness | - Risk of cracking |
- Enhanced toughness | - Additional processing steps |
Quenching Methods
In this section, we will explore various techniques used to rapidly cool alloys in order to enhance their strength and durability. The process of quenching involves subjecting a heated material to a sudden change in temperature, typically by immersing it in a cooling medium. By employing specific quenching methods, engineers can tailor the properties of iron-based alloys to suit specific applications, resulting in improved structural integrity.
One commonly used quenching method is water quenching, where the heated alloy is rapidly cooled by immersion in water. This method offers a quick cooling rate, allowing for the formation of a fine-grained microstructure, which contributes to increased strength and hardness. Another popular technique is oil quenching, where the alloy is submerged in oil instead of water. Oil provides a slower cooling rate compared to water, yielding a different microstructure and properties. The choice between water and oil quenching depends on the desired characteristics of the final product.
Another technique, known as air quenching, involves exposing the heated alloy to ambient air. This method relies on convection to cool the material gradually, resulting in a more uniform cooling rate. Air quenching is often used for less critical applications, where the highest levels of strength and hardness are not required. On the other hand, salt quenching utilizes a salt bath to cool the material. This technique offers precise control over the cooling rate and is commonly employed for specialized alloys.
Additionally, some alloys benefit from a two-step quenching process called double quenching. This involves first quenching the material in a medium like water or oil, followed by a subsequent quench in a different medium. The combination of quenching methods helps to achieve specific properties and desired alloy structures.
In conclusion, quenching methods play a critical role in enhancing the strength and structural integrity of iron-based alloys. By carefully selecting the appropriate quenching technique and parameters, engineers can manipulate the microstructure and properties of alloys, ultimately resulting in materials that meet the demanding requirements of various industries.
Tempering Techniques
Exploring methods to enhance the strength and durability of iron components involves delving into the realm of tempering techniques. These innovative approaches focus on optimizing the structural integrity of iron while ensuring its longevity and resistance to various forms of stress.
One prominent tempering technique is stress relieving. By subjecting iron to controlled heating and cooling processes, stress within the material is alleviated, reducing the risk of structural failure. This technique allows for the iron to maintain its integrity and prevent the occurrence of cracks or fractures under heavy loads or extreme conditions.
Another valuable technique is precipitation hardening, which involves a two-step process: solution treatment and aging. During solution treatment, iron is heated to a specific temperature and alloy elements are dissolved. Subsequently, aging is employed to promote the formation of fine precipitates within the iron, resulting in increased strength and hardness. This technique effectively tunes the microstructure of iron to achieve the desired properties.
Additive manufacturing techniques, such as selective laser melting or electron beam melting, also offer potential for enhancing the structural integrity of iron. These techniques enable the precise control of cooling rates and the formation of distinct microstructures, leading to improved mechanical properties. Additionally, through the incorporation of specific alloying elements, the mechanical performance of iron components can be enhanced further, rendering them more durable and resistant to environmental factors.
Indeed, the exploration of tempering techniques continues to expand, uncovering novel approaches to strengthen iron and extend its lifespan. By harnessing the potential of stress relieving, precipitation hardening, and additive manufacturing, engineers and researchers are making significant strides towards optimizing the structural integrity of iron for diverse applications.
Alloying Elements
The incorporation of various alloying elements plays a crucial role in enhancing the strength and performance of iron alloys. By introducing different elements into iron, engineers and scientists have discovered innovative ways to optimize the structural integrity of iron-based materials. This section explores the key alloying elements that contribute to the improved properties of iron alloys, without relying on specific definitions.
- 1. Reinforcing Additives
One category of alloying elements used to strengthen iron alloys includes reinforcing additives. These substances are strategically added to the iron matrix to increase its hardness, durability, and resistance to deformation. Additionally, reinforcing additives help counterbalance the inherent weaknesses of iron, such as its susceptibility to corrosion and brittleness. Through careful selection and precise blending of reinforcing additives, engineers have been able to develop iron alloys with exceptional mechanical properties.
- 2. Enhancing Alloying Agents
Another group of alloying elements are classified as enhancing alloying agents. These elements possess unique properties that enable them to improve specific characteristics of iron alloys. Examples of enhancing alloying agents include elements that enhance corrosion resistance, heat resistance, and electrical conductivity. By incorporating these elements into iron alloys, engineers can tailor the material’s performance to meet the requirements of various applications, ranging from automotive manufacturing to aerospace engineering.
- 3. Modifying Elements
Modifying elements form another crucial class of alloying elements used in iron alloys. As the name suggests, these elements modify the microstructure of iron, altering its properties and behavior. Modifying elements can refine the grain structure of iron alloys, improve their machinability, and enhance their weldability. The strategic addition of these elements allows engineers to fine-tune iron alloys to achieve a desirable combination of strength, toughness, and formability, making them suitable for a wide range of structural applications.
In conclusion, alloying elements incorporated into iron alloys have revolutionized the field of materials engineering, enabling the development of stronger and more versatile structural materials. By carefully selecting and introducing reinforcing additives, enhancing alloying agents, and modifying elements, engineers can harness the full potential of iron alloys for various applications, ensuring enhanced structural integrity and improved overall performance.
Effect of Carbon Content
The impact of carbon content on the properties of iron is a crucial area of study in the quest for enhanced structural integrity. The presence of carbon in iron can significantly alter its strength, hardness, and ductility. Understanding and manipulating the carbon content within iron opens up opportunities for improving its overall performance in various applications.
Influence on Strength: The carbon atoms in iron form interstitial solid solutions, which create dislocations and strengthen the lattice structure. Higher carbon content leads to increased strength by enabling more dislocations to move through the material, improving its resistance to deformation and fracture. However, an overly high carbon content can also introduce brittleness, compromising the structural integrity.
Impact on Hardness: Carbon enhances the hardness of iron alloys by forming carbides, which are hard and brittle compounds. Higher carbon content results in the formation of more carbides, thereby increasing the hardness of the material. However, excessive carbon can lead to excessive carbide formation, which can make the alloy too brittle for practical use.
Effect on Ductility: The presence of carbon in iron can reduce its ductility, making it less capable of withstanding deformation without fracturing. Lower carbon content is generally preferred when high ductility is required. However, a moderate carbon content can improve the alloy’s ductility by reducing the grain size and promoting dislocation movement, striking a balance between strength and ductility.
Controlling Carbon Content: The carbon content in iron can be controlled during the manufacturing process by adjusting the composition of the alloy and employing various heat treatment techniques. Proper control of carbon content allows engineers to tailor the material to meet specific structural requirements, ensuring optimal performance in different load-bearing applications.
In conclusion, the carbon content plays a crucial role in determining the strength, hardness, and ductility of iron alloys. By understanding and effectively controlling the carbon content, engineers can enhance the overall structural integrity of iron, making it suitable for a wide range of applications that require robust and durable materials.
Alloying Elements for Strength
In the pursuit of enhancing the robustness of iron and its various alloys, researchers have explored the incorporation of specific elements to improve strength and durability. By combining iron with carefully selected alloying elements, engineers strive to develop materials capable of withstanding high stress loads and maintaining structural integrity. This section delves into the significance of alloying elements in the quest for stronger iron-based materials.
The addition of alloying elements introduces a range of unique properties that contribute to the overall strength of iron alloys. These elements not only enhance mechanical properties such as tensile strength and hardness but also play crucial roles in improving resistance to corrosion, fatigue, and creep. By carefully choosing the composition and concentration of alloying elements, engineers can tailor the iron- alloy to specific applications, ensuring optimal performance and longevity.
Alloying elements such as chromium have been widely utilized for their ability to improve corrosion resistance, particularly in stainless steels. Chromium forms a protective oxide layer on the surface of the alloy, safeguarding it against chemical attacks and preventing the formation of rust. Additionally, it enhances the alloy’s hardness, making it suitable for applications requiring resistance to wear and abrasion.
Manganese is another key alloying element that brings notable benefits to iron-based materials. It enhances strength and toughness, making it an excellent choice for structural applications. Manganese also acts as a deoxidizer, removing oxygen impurities during the alloying process, thereby preventing the formation of voids or weak spots in the material.
Nickel, often employed in conjunction with chromium, significantly enhances the mechanical properties of iron alloys. It provides excellent resistance to both corrosion and heat, making it an indispensable element in alloys used in extreme environments. Nickel also aids in maintaining stability at elevated temperatures, ensuring structural integrity even under high-stress conditions.
It is worth noting that this section provides only a glimpse into the vast array of alloying elements used in enhancing the strength of iron-based materials. Each element brings its own unique advantages and properties, allowing engineers to tailor iron alloys for specific applications and requirements.
Advanced Techniques
In this section, we will explore innovative and cutting-edge methodologies and approaches that are revolutionizing the field of enhancing the strength and durability of iron. These advanced techniques encompass a diverse range of processes and methodologies aimed at improving the overall performance and structural integrity of iron-based materials.
One key area of focus within advanced techniques is the utilization of novel fabrication methods that go beyond traditional manufacturing approaches. This includes additive manufacturing, also known as 3D printing, which allows for the creation of complex and customized iron structures with enhanced strength and integrity. Additionally, advanced machining techniques such as laser cutting and high-precision milling enable precise shaping and refining of iron components, resulting in improved structural properties.
Another aspect of advanced techniques is the use of advanced surface treatment technologies, such as plasma nitriding and ion implantation. These processes modify the surface properties of iron by introducing elements or changing the structure at the atomic level. This leads to improved resistance against wear, corrosion, and fatigue, thereby enhancing the overall structural integrity of iron materials.
Furthermore, advanced alloying methods play a crucial role in strengthening iron. By incorporating various elements into the iron matrix, alloys with enhanced mechanical properties can be obtained. Techniques like powder metallurgy, alloy optimization through computational modeling, and rapid solidification offer new avenues for developing high-performance iron alloys with improved strength, toughness, and resistance to environmental factors.
In conclusion, the exploration of advanced techniques in enhancing the strength of iron goes beyond conventional methods and opens up new possibilities for improving the structural integrity of iron materials. These innovations in fabrication, surface treatment, and alloying methods are vital in meeting the demands of modern applications requiring strong and durable iron structures.
Surface Modification Methods
The section on surface modification methods explores various techniques and processes that can be applied to improve the properties and characteristics of metallic surfaces. By utilizing a range of methods, engineers and scientists can enhance the performance, durability, and functionality of materials without compromising their structural integrity.
One approach to surface modification involves the use of coatings. Coatings can provide a protective barrier against corrosion, wear, and other environmental factors, thereby extending the lifespan of the material. Common coating methods include electroplating, thermal spraying, and chemical vapor deposition.
Another method is surface treatment, which involves altering the composition or structure of the surface to enhance specific properties. Heat treatment, for example, can improve hardness and strength by controlling the microstructure of the material. Shot peening is another technique that induces beneficial compressive stresses on the surface, increasing fatigue resistance. Additionally, ion implantation and laser surface modification can be used to enhance wear resistance and reduce friction.
To further improve the performance of metallic surfaces, surface engineering techniques can be employed. These techniques involve the addition of elements or compounds to the surface to create a desired effect. For instance, alloying can enhance mechanical properties, such as strength and toughness, by introducing alloying elements into the surface layer. Diffusion processes, such as carburizing and nitriding, can improve surface hardness and wear resistance by enriching the surface with carbon or nitrogen atoms.
In conclusion, surface modification methods offer a range of techniques and processes to enhance the properties and characteristics of metallic surfaces. Through the application of coatings, surface treatments, and surface engineering techniques, engineers and scientists can tailor the surface properties to meet specific performance requirements. These methods play a vital role in improving the overall performance and reliability of structural materials.
Nanotechnology Applications
Exploring the vast potential of nanotechnology in enhancing the properties and performance of materials.
Nanotechnology, with its ability to manipulate matter at the atomic and molecular scale, offers numerous possibilities for improving the strength and characteristics of materials. This section delves into the applications of nanotechnology in enhancing the structural integrity of various materials, without focusing specifically on the process of strengthening iron or exploring techniques and alloys.
Application | Description |
---|---|
Nanocoatings | By utilizing nanomaterials, coatings can be engineered to provide enhanced protection against corrosion, abrasion, and wear. |
Nanocomposites | Combining nanoparticles with conventional materials leads to the development of composite materials with improved strength, flexibility, and conductivity. |
Nanoparticle reinforcement | Nanoparticles can be incorporated into materials to reinforce their structure, increasing their resistance to deformation under stress. |
Nanofabrication techniques | Controlling the assembly of nanoscale structures allows for the creation of materials with tailored properties and precise engineering characteristics. |
Nanomanipulation | With advanced manipulation techniques, nanoscale structures can be carefully arranged to optimize material strength and performance. |
Self-assembled monolayers | Using molecular self-assembly, thin films can be created at the nanoscale to modify surface properties, such as adhesion and friction. |
These applications showcase the potential of nanotechnology in revolutionizing various industries, offering new opportunities for designing materials with superior structural integrity and enhanced performance.