"Iron's Impact on Monocyte-Macrophage Polarization Mechanisms and Implications"
Irons Impact on Monocyte-Macrophage Polarization Mechanisms and Implications: Understanding the role of iron in immune cell function, differentiation, and regulation, and its implications for health and disease.
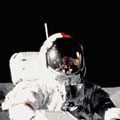
- Anthony Arphan
- 11 min read
The dynamic nature of our immune system relies heavily on the intricate interactions and transformations of various cell types. Among these, specific immune cells undergo significant changes in their function and state in response to diverse stimuli, a process crucial for maintaining health and combating diseases. One essential element that significantly affects these cellular transitions is a well-known mineral.
This vital nutrient plays a critical part in shaping the responses of immune cells, particularly those that are key players in inflammation and tissue repair. By modulating the activity and characteristics of these cells, the nutrient influences how our body responds to infections, injuries, and chronic conditions. The complex pathways through which this occurs have been the subject of extensive research, shedding light on the multifaceted ways our immune defenses are regulated.
Understanding the subtleties of this modulation is crucial for developing therapeutic strategies that can enhance immune function or mitigate adverse inflammatory responses. The interplay between the nutrient and immune cells not only reveals fundamental biological processes but also opens doors to potential interventions in a variety of health issues, ranging from autoimmune disorders to cancer. This article delves into these mechanisms, offering insights into how a simple dietary component can have profound implications for our immune health.
Iron Regulation in Immune Cell Function
The regulation of iron is crucial for the proper function of immune cells. This essential mineral is involved in various cellular processes, influencing the behavior and efficiency of immune responses. The delicate balance of iron levels within immune cells is necessary to maintain their health and functionality. Disruption in this balance can lead to impaired immune responses and increased susceptibility to infections or inflammatory conditions.
Iron’s influence on immune cells encompasses multiple dimensions, including cellular metabolism, proliferation, and differentiation. The following table provides a concise overview of the key functions and effects of iron within different types of immune cells.
Immune Cell Type | Key Functions | Impact of Iron Regulation |
---|---|---|
Neutrophils | First responders in inflammation, phagocytosis, and pathogen clearance | Iron availability enhances their bactericidal activity and survival |
T Lymphocytes | Cell-mediated immunity, including cytotoxic activity and helper functions | Iron levels affect their proliferation, differentiation, and cytokine production |
B Lymphocytes | Antibody production and adaptive immunity | Iron homeostasis is vital for their differentiation into plasma cells |
Macrophages | Phagocytosis, antigen presentation, and cytokine production | Iron balance influences their activation states and function in tissue repair and immunity |
Dendritic Cells | Antigen presentation and initiation of adaptive immunity | Iron modulation affects their maturation and ability to activate T cells |
Understanding the intricacies of iron regulation within immune cells offers insights into potential therapeutic approaches for managing immune-related disorders. By modulating iron levels, it may be possible to enhance immune responses or mitigate excessive inflammation, thereby improving overall health outcomes.
Monocyte to Macrophage Differentiation
The transformation of monocytes into macrophages is a complex and essential process within the immune system. This section will explore the stages of this transition, highlighting the cellular and molecular changes that occur. Understanding these mechanisms provides insight into the functioning of the immune response and its impact on health and disease.
Monocytes, a type of white blood cell, circulate in the bloodstream and are recruited to sites of infection or tissue damage. Upon arrival, they undergo a series of changes, maturing into macrophages. This differentiation is crucial for effective immune defense, as macrophages are key players in phagocytosis, antigen presentation, and the secretion of various cytokines and growth factors.
The differentiation process is influenced by various signals from the surrounding microenvironment, including cytokines, growth factors, and extracellular matrix components. These signals activate specific transcription factors and signaling pathways within the monocytes, guiding their maturation into macrophages. Additionally, the local tissue context can determine the functional phenotype of the resulting macrophages, tailoring their activity to meet the specific needs of the tissue.
Macrophages display remarkable plasticity, adapting to different functional states depending on the signals they receive. This adaptability allows them to perform diverse roles, from pro-inflammatory actions aimed at pathogen destruction to anti-inflammatory activities that promote tissue repair. The balance between these states is critical for maintaining homeostasis and responding appropriately to various challenges.
In summary, the differentiation of monocytes into macrophages is a dynamic and finely regulated process. It is essential for the proper functioning of the immune system, enabling a tailored response to a wide range of stimuli. By understanding the factors that drive this differentiation, researchers can better grasp the intricacies of immune regulation and the potential for therapeutic interventions in immune-related diseases.
Iron Metabolism in Macrophages
Understanding how this essential mineral is processed within macrophages is crucial for comprehending their functionality in various physiological contexts. This section delves into the pathways and processes involved in the absorption, storage, and utilization of this critical element within these immune cells.
Macrophages are central to maintaining mineral balance in the body. They acquire this element through various mechanisms, such as receptor-mediated endocytosis of transferrin-bound ferrum or phagocytosis of erythrocytes. Once internalized, it is stored in ferritin or utilized for essential cellular functions. The precise regulation of these pathways ensures that macrophages can effectively respond to environmental stimuli and participate in immune responses.
Process | Description |
---|---|
Acquisition | Macrophages obtain ferrum through the uptake of transferrin-bound ferrum and erythrophagocytosis. |
Storage | The mineral is stored in ferritin within the macrophages to maintain homeostasis and prevent toxicity. |
Utilization | Stored ferrum is utilized for cellular processes, including DNA synthesis, electron transport, and as a cofactor for various enzymes. |
Export | Excess ferrum is exported out of macrophages through ferroportin to maintain systemic balance. |
The regulation of this mineral’s metabolism in macrophages is influenced by various factors, including inflammatory signals, which can alter the expression of key proteins involved in its uptake, storage, and release. For instance, inflammatory cytokines can induce hepcidin production, which inhibits ferroportin, reducing mineral export and increasing intracellular ferrum levels.
This dynamic regulation allows macrophages to adapt to changing physiological conditions, playing a pivotal role in both local tissue environment and systemic mineral homeostasis. Understanding these processes is essential for developing therapeutic strategies to modulate macrophage function in various diseases.
Iron Uptake Mechanisms
The process by which cells acquire iron is fundamental to their function and survival. This section delves into the various pathways and methods through which iron is absorbed and internalized by cells, emphasizing the complexity and significance of these processes in maintaining cellular health and function.
Transferrin-Mediated Endocytosis is one of the primary methods through which cells acquire iron. In this pathway, iron binds to the glycoprotein transferrin, which then interacts with transferrin receptors on the cell surface. The iron-transferrin complex is subsequently internalized through endocytosis. Inside the cell, the acidic environment of the endosome facilitates the release of iron from transferrin, allowing it to be utilized by the cell while transferrin is recycled back to the cell surface.
Another significant pathway is non-transferrin-bound iron (NTBI) uptake. This mechanism becomes especially important in conditions where the levels of iron exceed the binding capacity of transferrin, leading to the presence of free iron in the bloodstream. Cells can internalize this excess iron through a variety of transporters, including divalent metal transporter 1 (DMT1) and other members of the solute carrier family. This pathway helps to ensure that cells can still acquire necessary iron even when transferrin-mediated uptake is saturated.
The role of ferritin in iron uptake is also crucial. Ferritin, an iron-storage protein, can sequester iron within its hollow shell, thus preventing free iron from catalyzing the formation of harmful free radicals. Cells can acquire iron by internalizing ferritin through receptor-mediated endocytosis. Once inside the cell, ferritin is broken down in lysosomes, releasing the stored iron for cellular use.
Furthermore, cells utilize heme-iron uptake mechanisms, particularly relevant for macrophages involved in the recycling of red blood cells. Heme, the iron-containing component of hemoglobin, is absorbed by macrophages through specific heme transporters such as heme carrier protein 1 (HCP1). The internalized heme is then degraded by heme oxygenase enzymes, releasing iron for the cell’s metabolic needs.
Understanding these diverse and intricate iron uptake processes highlights the essential nature of iron homeostasis in cellular physiology, shedding light on how disruptions in these pathways can lead to various disorders and diseases.
Storage and Release Processes
Understanding the intricate balance between accumulation and liberation of certain elements within immune cells is crucial for comprehending their functional dynamics. This section delves into the ways these cells manage their internal reservoirs, as well as the triggers and pathways that facilitate the mobilization of stored materials.
The storage of elements within these cells is a finely tuned process, ensuring a ready supply for various cellular activities. The mechanisms of sequestration involve specific proteins and organelles designed to maintain optimal levels and prevent toxicity. Here are some key aspects of this process:
- Sequestration: Specialized proteins and organelles that capture and store essential elements.
- Regulation: Cellular systems that monitor and control the internal levels, ensuring balance and preventing overload.
- Protection: Mechanisms that shield the cell from potential damage caused by excess accumulation.
The release of these stored materials is equally critical, often dictated by cellular signals and environmental cues. This release supports various physiological functions, including immune responses and tissue repair. The following points highlight key factors in this release process:
- Signals: Internal and external stimuli that initiate the mobilization of stored elements.
- Pathways: Specific routes within the cell through which the stored materials are transported to their sites of action.
- Effects: The immediate and long-term impacts of the release on cellular activities and overall function.
By exploring these storage and release mechanisms, we gain valuable insights into how cells maintain homeostasis and respond to various physiological demands.
Impact of Iron on Macrophage Polarization
The influence of iron on the behavior of immune cells, particularly macrophages, is a significant area of study. Understanding how iron modulates these cells’ functions can provide valuable insights into various physiological and pathological processes. This section explores how different levels of iron can affect the activity and characteristics of macrophages, potentially leading to diverse immune responses.
Macrophages, known for their versatility in the immune system, can adopt different functional states in response to various stimuli. These states, often described as “classical” or “alternative,” reflect the macrophages’ capacity to either promote inflammation or facilitate tissue repair. The availability of iron is a crucial factor that can sway macrophages towards one state or another, impacting their role in immune regulation.
Iron availability affects macrophage activation and their subsequent actions in the body. When iron levels are high, macrophages are more likely to adopt a pro-inflammatory phenotype. This state is characterized by the production of inflammatory cytokines and an enhanced ability to combat pathogens. Conversely, low iron conditions tend to drive macrophages towards an anti-inflammatory phenotype, which supports tissue repair and resolution of inflammation.
At the molecular level, iron can influence the expression of various genes and proteins within macrophages. This modulation can alter their metabolism, signaling pathways, and functional responses. For instance, iron can affect the activity of transcription factors such as NF-κB and STAT3, which play crucial roles in determining macrophage behavior.
Furthermore, the iron status of macrophages can impact their interaction with other cells and tissues. For example, in the context of chronic diseases such as cancer or autoimmune disorders, the iron-induced polarization of macrophages can either exacerbate disease progression or aid in recovery, depending on the context.
In conclusion, the availability and regulation of iron are pivotal in shaping the functional landscape of macrophages. By influencing their activation states, iron can modulate immune responses, offering potential therapeutic targets for managing various inflammatory and chronic conditions.
Pro-Inflammatory (M1) Activation
The transformation of immune cells into a state that enhances inflammatory responses is a critical aspect of the body’s defense mechanism. This shift results in cells that are adept at tackling pathogens and initiating strong immune reactions. The intricate processes driving this shift are of immense interest in understanding how the immune system responds to various stimuli and how it can be modulated for therapeutic purposes.
During the M1 activation phase, several signaling pathways are triggered, leading to a cascade of events that enhance the cells’ pro-inflammatory characteristics. This includes increased production of reactive oxygen species (ROS), upregulation of surface markers, and secretion of pro-inflammatory cytokines. These cellular changes are crucial for mounting an effective response against infections and other immune challenges.
Characteristic | Description |
---|---|
Surface Markers | Enhanced expression of molecules such as CD80, CD86, and MHC class II, which are essential for antigen presentation and T-cell activation. |
Cytokine Production | Increased secretion of pro-inflammatory cytokines like TNF-α, IL-6, and IL-12, which play a pivotal role in mediating immune responses. |
Reactive Oxygen Species (ROS) | Elevated levels of ROS, contributing to microbial killing and signaling functions within the immune system. |
Understanding the specific triggers and regulators of this activation state is crucial for developing strategies to manipulate immune responses in diseases characterized by chronic inflammation or impaired immune function. Research in this area continues to unveil the complexities of immune cell behavior and offers potential avenues for therapeutic interventions.
Anti-Inflammatory (M2) Activation
The process of transforming immune cells towards a healing state involves complex signaling and environmental interactions. This transition is crucial for resolving inflammation and promoting tissue repair. Understanding these mechanisms provides insights into potential therapeutic approaches for various inflammatory conditions.
Key aspects of this transformation include:
- Signaling Pathways: Specific biochemical signals trigger the shift towards a healing phenotype, influencing the behavior and function of immune cells.
- Cytokine Influence: Certain proteins play a pivotal role in guiding cells towards an anti-inflammatory state, aiding in the suppression of harmful immune responses.
- Metabolic Changes: Alterations in cellular metabolism are essential for supporting the energy requirements and functions of healing cells.
- Environmental Factors: The surrounding microenvironment significantly impacts the activation and maintenance of the healing phenotype.
These factors collectively ensure that immune cells adopt a state conducive to reducing inflammation and fostering recovery. This understanding paves the way for developing strategies to harness this activation in therapeutic contexts.