Unraveling the Mysteries of Cosmic Iron - The Significance of Iron in Astrophysics and its Exploration in Outer Space
Iron in Space: Understanding the Cosmic Iron and its Role in Astrophysics and Space Exploration
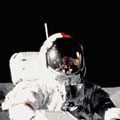
- Anthony Arphan
- 23 min read
Iron, one of the most abundant elements in the universe, plays a crucial role in astrophysics. From the depths of space to the heart of stars, iron holds the key to understanding the enigmas of our cosmic environment. Its unique properties and ubiquitous presence make it a fascinating subject for study, as scientists strive to unravel its mysteries.
Iron is not only found on Earth; it is pervasive throughout the universe. It is forged in the cores of massive stars through the process of nuclear fusion, where lighter elements are transformed into heavier ones, eventually leading to the creation of iron. When these stars explode in supernovae, they scatter iron-rich stardust across the cosmos, seeding new stars and planets.
The presence of iron in space serves as a cosmic time capsule, providing valuable insights into the history and evolution of our universe. By studying the abundance and distribution of iron in different astronomical objects, such as galaxies, nebulae, and even interstellar dust clouds, scientists can gain a deeper understanding of the processes that have shaped our cosmic environment over billions of years.
Iron has also proven to be a powerful tool for probing the vast distances of space. Its spectral lines, which are unique fingerprints of iron atoms, can be observed in the light emitted by distant celestial objects. By analyzing these spectral lines, astronomers can determine vital information about the object’s composition, temperature, and motion, allowing them to study celestial bodies that are billions of light-years away.
In addition to its importance in astrophysics, iron has practical applications here on Earth. It is a critical component in the construction of telescopes and satellites, as its high melting point and durability make it ideal for withstanding the harsh conditions of space. Furthermore, the study of iron in space has implications for understanding and developing new materials and technologies on Earth.
As our exploration of space continues to unfold, the enigmas of cosmic iron will undoubtedly continue to captivate the minds of scientists and researchers. Its significance in shaping the universe we inhabit, its role in the evolution of stars and galaxies, and its potential applications in technology make it a subject of endless fascination and promise. The study of iron in space is not just about understanding the past and present, but also about unlocking the mysteries of the future.
The Mysteries of Iron in Space
Iron, despite being one of the most common elements on Earth, holds a profound significance in the vast expanse of space. Its presence in celestial bodies and interstellar matter has puzzled astronomers and astrophysicists for decades, as its origins and role in astrophysical processes remain enigmatic.
One of the main enigmas surrounding iron in space is its abundance. Although iron is the heaviest element that can be formed through nuclear fusion in the core of massive stars, its presence in distant galaxies and young astronomical objects is surprisingly high. Scientists speculate that iron might have been produced through other mechanisms, such as high-energy stellar collisions or byproducts of supernova explosions.
Another mystery is the distribution of iron in the universe. Observations have revealed that iron is not evenly distributed within galaxies, but rather concentrated in specific regions, such as star-forming regions or galactic nuclei. Understanding why iron is preferentially located in these areas can provide crucial insight into the processes that govern the formation and evolution of galaxies.
Furthermore, iron plays a vital role in astrophysical phenomena, such as the formation of stars and planets. Iron-rich dust grains serve as catalysts for the formation of molecular hydrogen, a key ingredient in star and planet formation. Investigating the conditions and mechanisms that lead to the formation of iron-rich dust is essential for comprehending the intricate processes that shape the cosmos.
Moreover, iron is a fundamental component of stellar evolution. Throughout a star’s lifetime, iron acts as a catalyst in nuclear reactions, fueling the fusion of lighter elements and releasing energy. The understanding of iron’s behavior and its role in stellar evolution can enhance our knowledge of the life cycles of stars and the processes that govern their deaths.
Overall, the mysteries surrounding iron in space are captivating and offer an abundance of opportunities for scientific exploration. They drive researchers to unravel the origins, distribution, and role of iron in astrophysical phenomena, shedding light on the enigmas of the universe.
Understanding the Cosmic Iron
Iron, often referred to as the “building block” of the universe, plays a crucial role in astrophysics. It is one of the most abundant elements found in stars, supernovae remnants, and interstellar gas clouds. Understanding the cosmic iron is essential for unraveling the mysteries of the universe.
Iron is created through stellar nucleosynthesis, the process by which elements are formed in the cores of stars. During the late stages of a star’s life, fusion reactions occur, turning lighter elements into heavier ones. These fusion reactions generate immense amounts of energy, and as a result, iron is the heaviest element that can be produced in a star’s core.
Iron is also present in meteorites and asteroids, which provide valuable insights into the early stages of our solar system’s formation. By studying these extraterrestrial bodies, scientists can gain a better understanding of how iron and other elements were distributed and incorporated into planets.
The presence of iron in a star can have a significant impact on its evolution. Iron acts as a catalyst for the fusion reactions that power stars. When a star runs out of lighter elements to fuse, it begins to burn iron in a process known as iron burning. This stage signifies the end of the star’s life and leads to a supernova explosion, dispersing iron and other elements into the surrounding space.
Iron is also an important component of interstellar dust, which plays a crucial role in the formation of new stars and planets. The presence of iron-rich dust grains can facilitate the condensation and aggregation of other elements, leading to the formation of protoplanetary disks and ultimately planetary systems.
Understanding the abundance and distribution of cosmic iron is vital for studying the evolution of galaxies. Iron can act as a tracer of past supernova explosions and the chemical enrichment of galaxies over time. By analyzing the iron content in distant galaxies, astronomers can gain insights into the formation and evolution of these cosmic structures.
In conclusion, the study of cosmic iron is essential for deepening our understanding of the universe. From its role in stellar nucleosynthesis to its presence in meteorites and interstellar dust, iron provides valuable clues about the origin and evolution of celestial bodies. By unraveling the enigmas of cosmic iron, scientists can unlock the secrets of the cosmos.
Formation of Iron in Supernovae
Supernovae play a crucial role in the formation of iron in the Universe. These explosive events occur when massive stars reach the end of their lives and undergo a catastrophic collapse. During the collapse, the core of the star becomes extremely dense and hot, triggering a runaway nuclear fusion process.
As the fusion reactions progress, elements heavier than helium are synthesized. However, the fusion process is unable to produce elements heavier than iron. This is because iron has the highest binding energy per nucleon, making it the most stable nucleus. Any further fusion reactions would require more energy than they release.
When the collapsing core of a massive star reaches a critical density, it undergoes a rapid implosion, resulting in a supernova explosion. The extreme temperatures and pressures generated during the explosion allow for the formation of even heavier elements, including iron.
During the explosive phase of a supernova, shock waves propagate through the stellar material, causing rapid compression and heating. This compression and heating allow lighter elements, such as carbon and oxygen, to fuse into heavier elements like silicon and sulfur. Eventually, the shock wave reaches the iron core of the star, leading to the rapid production of iron and its isotopes.
The abundance of iron produced in supernovae is essential for the evolution of galaxies and the enrichment of the interstellar medium. The iron ejected during a supernova explosion seeds the surrounding gas clouds, providing the raw materials for further star formation. Over time, subsequent generations of stars form from these enriched gas clouds, ensuring that iron remains a common element throughout the Universe.
Studying the formation of iron in supernovae helps astrophysicists understand the life cycles of stars, the nucleosynthesis processes, and the chemical evolution of galaxies. By analyzing the spectra of supernova remnants and studying the distribution of iron in the Universe, scientists can trace the history of iron production and track the cosmic recycling of elements.
In conclusion, the formation of iron in supernovae is a fascinating process that contributes to the rich tapestry of the Universe. This enigmatic element is not only vital for understanding the physics of stellar evolution but also for unraveling the mysteries of the cosmos.
Role of Neutron Stars in Iron Synthesis
The formation of iron in space is a captivating puzzle that has intrigued astrophysicists for decades. While the fusion of lighter elements in the hearts of massive stars is a well-understood process, the production of iron poses a unique challenge. It is through the cataclysmic events involving neutron stars that this enigma is unraveled.
Neutron stars, which are the collapsed remnants of massive stars, play a crucial role in the synthesis of iron. These incredibly dense objects possess a rapid rotation, emitting beams of electromagnetic radiation like cosmic lighthouses. These beams can be observed as pulsating signals, hence the name pulsars.
During the formation of a neutron star, the intense pressure and heat lead to the degeneracy of electrons and protons. The protons and electrons combine to form neutrons, resulting in a mass of neutrons densely packed together. This dense environment provides the ideal conditions for the process known as rapid neutron capture, or the r-process.
In the r-process, neutrons are rapidly captured by atomic nuclei, creating heavier and heavier elements. Eventually, this process reaches the formation of iron, which is the most stable nucleus with the highest binding energy per nucleon. The iron synthesis occurs in the extreme conditions near the surface of a neutron star, where the tremendous gravitational forces and temperatures drive the r-process to its endpoint.
The iron produced in neutron stars plays a vital role in astrophysics. As neutron stars age, they eventually reach a critical point where the pressure from the electrons balancing the gravitational forces becomes too great. At this point, a supernova explosion occurs, dispersing the newly synthesized iron into the interstellar medium.
This iron is then incorporated into future generations of stars, providing the building blocks for the formation of rocky planets like Earth. Moreover, the ejected iron contributes to the enrichment of interstellar gas, which in turn influences the composition of galaxies and the evolution of the universe.
The role of neutron stars in iron synthesis is a fascinating phenomenon that highlights the intricate interplay between the life cycles of stars and the cosmic elements they produce. Further studies and observations of neutron stars will continue to shed light on this extraordinary process, advancing our understanding of the enigmas of cosmic iron and its importance in astrophysics.
Exploring Iron-Rich Celestial Bodies
Iron, one of the most abundant elements in the universe, plays a crucial role in the formation and evolution of celestial bodies. From stars to galaxies, iron can be found in various forms, and its presence can provide valuable insights into the cosmological processes that shape our universe.
Iron-rich celestial bodies, such as iron meteorites or iron-nickel asteroids, offer unique opportunities for astronomers and astrophysicists to study the origins and composition of these extraterrestrial objects. By analyzing the isotopic ratios of iron in these celestial bodies, scientists can gain a better understanding of the processes that led to their formation, including nucleosynthesis and stellar explosions.
Iron is not only important for understanding the history of celestial bodies but also has practical implications for space exploration. The presence of iron on celestial bodies like the Moon or Mars could potentially be exploited for future human colonization efforts. Iron could be extracted from these bodies and used for building structures, manufacturing tools, or even producing oxygen for astronauts.
Furthermore, the study of iron in space can provide insights into the broader astrophysical processes that govern the evolution of galaxies and the formation of stars. Iron is created through nuclear fusion in the core of massive stars and is dispersed into space during supernova explosions. By studying the distribution and abundance of iron in galaxies, scientists can piece together the cosmic history of star formation and the life cycles of galaxies.
In conclusion, exploring iron-rich celestial bodies is crucial for unravelling the mysteries of our universe. Through detailed analysis and investigation, scientists can gain valuable insights into the formation, composition, and evolution of these extraterrestrial objects. Moreover, the study of iron in space has both practical applications for future space exploration and broader implications for understanding the fundamental processes that shape the cosmos.
Iron in Asteroids and Meteorites
Asteroids and meteorites are remnants of the early solar system, and they provide valuable insights into the composition and evolution of our cosmic neighborhood. Iron is a common element found in asteroids and meteorites, and its presence can help scientists understand the processes that shaped the solar system.
Iron in asteroids is often found in the form of metallic iron-nickel alloys, commonly known as meteoric iron. These alloys can be up to 30% nickel, and they are thought to have formed through volcanic activity in the early solar system. Iron-rich asteroids, known as M-type asteroids, are believed to be some of the most primitive objects in our solar system, largely unchanged since their formation over 4.6 billion years ago.
Studying iron in asteroids and meteorites can provide insights into the formation and differentiation of planetary bodies. The presence of iron on the surface of asteroids, as well as within their interiors, can help scientists understand how these bodies formed and how they have evolved over time. By analyzing the isotopic composition of iron in meteorites, scientists can also determine the age of these objects and gain information about the processes that occurred during their formation.
Iron is also of interest in asteroids and meteorites due to its potential economic value. Iron-nickel alloys found in certain asteroids, such as the metallic asteroid Psyche, could be a rich source of valuable metals for future space mining endeavors. Understanding the distribution and abundance of iron in asteroids can inform future missions and technologies aimed at asteroid mining.
Overall, the study of iron in asteroids and meteorites plays a crucial role in our understanding of the early solar system and the processes that have shaped our cosmic environment. By analyzing the properties and composition of iron in these objects, scientists can unravel the mysteries of our celestial neighbors and gain insights into the formation and evolution of the planets, including our own Earth.
Iron in Exoplanets
Iron is an essential element in the universe, and its presence in exoplanets provides valuable insights into the formation and evolution of these celestial bodies. Exoplanets, or planets that orbit stars outside of our solar system, come in a wide range of sizes and compositions. By studying the iron content in exoplanets, scientists can gain a better understanding of their internal structure and properties.
One way to study iron in exoplanets is through transit spectroscopy, a method that involves measuring the changes in a star’s light as an exoplanet passes in front of it. This technique can reveal the presence of iron in the exoplanet’s atmosphere, which can be used to infer its composition and temperature.
Iron is particularly interesting in exoplanets because it can exist in different forms depending on the planet’s conditions. For example, in a hot and rocky exoplanet, iron may be present in a liquid or molten state, while in a cooler and more gaseous planet, it may be found in a solid or crystalline state. By studying the presence of different forms of iron in exoplanets, scientists can learn about the temperature, pressure, and chemical environment of these distant worlds.
The presence of iron in exoplanets also has important implications for their potential habitability. Iron is a key component in the formation of planetary cores, which are believed to be crucial for maintaining a stable magnetic field. A magnetic field is important for protecting a planet from harmful stellar radiation and for retaining its atmosphere. Therefore, understanding the iron content in exoplanets can provide insights into their habitability.
In conclusion, studying iron in exoplanets is a valuable endeavor that can shed light on the formation, composition, and habitability of these celestial bodies. By using techniques like transit spectroscopy, scientists can gain insights into the presence and state of iron in exoplanets, providing a deeper understanding of the vast diversity of planets beyond our solar system.
Iron’s Impact on Stellar Evolution
Iron plays a crucial role in the evolution of stars, as it is a key element in nuclear reactions that power the life cycles of these celestial bodies.
During the core fusion process in massive stars, hydrogen is converted to helium, and helium is further converted to heavier elements, including carbon and oxygen. Eventually, after several fusion stages, iron is produced in the core of a star.
Once a star’s core has a significant amount of iron, it can no longer sustain nuclear reactions. This leads to the collapse of the core and the subsequent explosive event known as a supernova. During a supernova, enormous amounts of energy are released, and heavy elements, including iron, are dispersed into space, enriching the surrounding interstellar medium.
Iron is also responsible for the formation of neutron stars and black holes. In the aftermath of a supernova, the core of the star may collapse into a dense neutron star composed primarily of iron atoms. In the most extreme cases, the core collapse can result in the formation of a black hole, where the gravitational pull is so strong that not even light can escape.
The presence of iron in space is not limited to supernovae remnants. Iron-rich stars, known as iron stars or hyper-iron stars, have been observed. These stars have an unusually high iron content, which may indicate a unique evolutionary path or the accretion of iron-rich material from their surroundings.
Studying iron in space provides valuable insights into the life cycles and dynamics of stars. By understanding the role of iron in stellar evolution, astronomers can better comprehend the processes that shape the universe and its galaxies.
Iron as a Stellar Spectroscopic Tool
Iron, the element with atomic number 26, holds significant importance in the field of astrophysics as a spectroscopic tool. Spectroscopy, the analysis of the interaction between matter and electromagnetic radiation, allows scientists to study the chemical composition, temperature, and velocity of astronomical objects.
Iron is particularly valuable in stellar spectroscopy due to its abundance and numerous absorption lines in the stellar spectra. These absorption lines, caused by the absorption of specific wavelengths of light by iron atoms, provide detailed information about the properties of stars.
By studying the iron absorption lines in a star’s spectrum, astronomers can determine its temperature, surface gravity, and metallicity. Temperature is inferred from the strength of the absorption lines, while surface gravity is derived from the line widths. Metallicity, or the abundance of elements heavier than hydrogen and helium, can be calculated by comparing the strength of iron absorption lines to other elements.
Iron also plays a crucial role in understanding stellar evolution. As stars age, they undergo fusion processes in their cores, converting hydrogen to helium and eventually fusing helium into heavier elements. Iron is a key element in this process as it acts as a catalyst to trigger supernova explosions, dispersing heavy elements into space for future generations of stars to form.
Furthermore, iron spectroscopy aids in the classification of stars into different spectral types and luminosity classes. The presence and characteristics of iron lines allow for the identification and categorization of stars, providing insights into their evolutionary stage, mass, and atmospheric properties.
In summary, iron’s presence in stellar spectra and its absorption lines provide invaluable information about the composition, temperature, gravity, and evolutionary stage of stars. It serves as a powerful tool for astronomers to unravel the mysteries of the universe and understand the processes that shape the cosmos.
Iron’s Influence on Star Formation
Iron plays a crucial role in the process of star formation, both in the formation of the initial protostar and throughout its evolution into a fully-fledged star.
During the collapse of a molecular cloud, where stars are born, iron acts as a catalyst for the fragmentation of the cloud. As the cloud collapses under its own gravity, iron-rich dust grains become heated, releasing thermal energy that counteracts the gravitational force. This thermal energy allows the cloud to resist further collapse and promotes the emergence of dense regions within the cloud, eventually leading to the formation of protostellar cores.
Once the protostar begins to form, iron continues to play a vital role. Iron atoms and ions are abundant in the gas surrounding the protostar, and they have a significant impact on the accretion process. As material from the surrounding cloud falls onto the protostar, some of it interacts with the iron atoms, triggering chemical reactions that release additional thermal energy. This energy not only provides the necessary heat for the protostar to continue growing but also helps expel excess material, preventing the protostar from becoming too massive.
As the protostar evolves into a main-sequence star, iron remains critically important. Nuclear reactions within the star’s core generate energy by fusing lighter elements into heavier ones, with iron being the heaviest element that can be produced through these reactions. Once a star begins to synthesize iron, it signals the end of its life as a typical main-sequence star and the beginning of the fusion of heavier elements and supernova explosion.
In summary, iron’s influence on star formation is multifaceted. It acts as a catalyst during the collapse of a molecular cloud, promotes the accretion process, and ultimately determines a star’s evolution and fate. Understanding the role of iron in star formation is crucial for unraveling the mysteries of the cosmic iron and its importance in astrophysics.
Iron as a Clue to the Universe’s Age and Origin
Iron, one of the most abundant elements in the universe, holds important clues about the age and origin of the cosmos. By studying the iron content in various celestial objects, scientists can gain insights into the formation and evolution of galaxies, stars, and planetary systems.
Iron is formed in the intense heat and pressure of supernovae explosions, where massive stars reach the end of their lives. These explosions scatter iron-rich material into space, and over time, this iron becomes incorporated into new stars and planetary systems. By analyzing the iron abundance in different regions of the universe, astronomers can determine the age of these objects and gain a better understanding of their formation processes.
Iron is also a key component of cosmic dust, tiny particles that exist in the interstellar medium. These dust particles play a crucial role in the formation of stars and planets, as they provide the building blocks for the formation of solid bodies. By studying the composition of cosmic dust, scientists can trace the evolution of these particles and gain insights into the origin of our own solar system.
Furthermore, iron is a crucial element in the study of the cosmic microwave background (CMB). The CMB is the residual radiation from the Big Bang that permeates the entire universe. By analyzing the iron abundance in the CMB, scientists can gather information about the early universe, such as its temperature and density. This data can then be used to estimate the age of the universe and support theories about its origin and evolution.
In conclusion, iron holds valuable clues about the age and origin of the universe. By studying the iron content in various celestial objects, scientists can unravel the mysteries of cosmic formation and gain a deeper understanding of our place in the vastness of space.
Iron Abundance in Primitive Stars
Understanding the abundance of iron in primitive stars is a crucial aspect of astrophysics. These ancient stars provide important insights into the early universe, as they formed shortly after the Big Bang. By studying the iron abundance in these stars, scientists can gather information about the processes that occurred during the early stages of stellar evolution.
Measuring the iron abundance in primitive stars is a challenging task. Since these stars are extremely metal-poor, traditional spectroscopic techniques often fail to provide accurate results. However, advancements in technology have enabled astronomers to develop new methods for determining the iron abundance in these stars. One such method is high-resolution spectroscopy, which allows for precise measurements of the iron lines in the stellar spectra.
By analyzing the iron abundance in primitive stars, scientists can gain insights into various astrophysical processes. For instance, the iron abundance can help determine the conditions present in the early universe, such as the density of matter or the rate of star formation. Additionally, studying the iron abundance in primitive stars can shed light on stellar evolution and nucleosynthesis, which are the processes by which elements are formed within stars.
The iron abundance in primitive stars also has implications for the study of galactic evolution. As iron is released through supernovae explosions, analyzing its abundance in primitive stars can provide information about the history of supernovae in our galaxy. This, in turn, can contribute to our understanding of how galaxies evolve and how the elements necessary for life are distributed throughout the universe.
Pros of studying iron abundance in primitive stars: | Cons of studying iron abundance in primitive stars: |
---|---|
- Provides insights into the early universe | - Challenging to measure accurately due to low metallicity |
- Helps determine conditions in the early universe | - Requires advanced spectroscopic techniques |
- Sheds light on stellar evolution and nucleosynthesis | |
- Provides information about galactic evolution |
In conclusion, studying the iron abundance in primitive stars is a significant aspect of astrophysics. It allows scientists to gain insights into the early universe, stellar evolution, and galactic evolution. Despite the challenges involved, advances in technology have enabled accurate measurements of the iron abundance in these stars, contributing to a comprehensive understanding of the universe and its history.
Iron as a Cosmic Chronometer
Iron, a common element found in the universe, plays a crucial role in determining the age of celestial bodies. Through its nuclear fusion and decay processes, iron isotopes provide valuable information about the chronology of astrophysical events.
Iron-56, the most abundant isotope of iron, is produced during the late stages of stellar evolution in massive stars. It is formed through nuclear reactions within the star’s core, where helium fuses to form carbon and oxygen, and eventually fuses into iron. This process marks the end of the star’s life, as it cannot generate energy from fusion reactions anymore.
Iron-60 is another isotope of iron that is of great interest to astrophysicists. Unlike iron-56, which is created within stars, iron-60 is primarily synthesized through nuclear reactions during supernovae explosions. These powerful stellar events release iron-60 into space, allowing scientists to study the age and occurrence of supernovae by analyzing the abundance of iron-60 residues in cosmic dust and meteorites.
The half-life of iron-60 is approximately 2.6 million years, which means that after this time period, only half of the original amount of iron-60 remains. By measuring the ratio of iron-60 to stable iron isotopes, researchers can estimate the age of supernovae remnants and the formation of our solar system. This cosmic chronometer allows us to unravel the mysteries of our universe and understand its evolution over time.
Furthermore, iron isotopes can also shed light on the cosmic origins of Earth and other planetary bodies. Primitive meteorites, known as chondrites, contain iron isotopes with excesses of iron-60 relative to iron-56, indicating their formation in the early solar system when the abundance of iron-60 was higher. These isotopic signatures provide insights into the composition and history of our solar system, as well as the processes that led to the formation of our planet.
In conclusion, iron isotopes serve as cosmic chronometers, allowing scientists to determine the age of astrophysical events and the formation of celestial bodies. Through the study of iron in space, we gain a better understanding of the evolution and history of our universe and our place within it.