Unveiling the Abundance and Environmental Consequences of Iron in the Natural World.
Iron in the Environment: Understanding its Presence, Effects, and Management Strategies
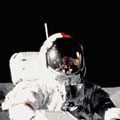
- Anthony Arphan
- 35 min read
Within the realms of natural ecosystems, an intricate network of elements and compounds shape the delicate balance of life. A crucial element that permeates the very fabric of nature, iron, stands steadfast as a fundamental building block. This mighty metal manifests itself in a myriad of forms, intricately entwined within the intricate web of life, embodying both strength and vulnerability. Today, we embark on a journey to explore the bountiful existence and profound ecological implications of iron in our natural surroundings.
From the rusted hues of ochre cliff faces to the vibrant crimson of blood coursing through living organisms, iron leaves its indelible mark on the canvas of nature. Not merely an inert mineral, iron possesses an innate duality that embraces both the gentle touch of support and the unyielding force of transformation. As natural processes unfurl, iron compounds play a role in the vital cycles of oxygen, energy production, and nutrient transportation. Its diverse roles extend from cofactors in enzymatic reactions to the intricate molecular machinery responsible for oxygen transport in hemoglobin.
The dance of iron within the spheres of nature is not confined to terrestrial ecosystems alone. Beneath the vast cerulean expanse of our oceans, iron pervades the watery depths, shaping the delicate balance that sustains marine life. From the microscopic diatoms that harness its energy for photosynthesis to the majestic whales that traverse its boundless expanses, iron’s influence on the intricacies of the marine realm is profound. Additionally, iron’s interactions with other elements give rise to stunning phenomena such as the mesmerizing red tide blooms, imparting a visual spectacle to the world beneath the waves.
However, despite its ubiquity and significance, iron also wields the potential for environmental impact. Human activities, from industrial processes to mining operations, can introduce excessive quantities of iron into delicate ecosystems, disturbing their equilibrium and jeopardizing the well-being of countless species. Understanding the intricate interplay between iron, its natural abundance, and its potential ecological consequences is vital to safeguarding the delicate web of life that depends upon it. As we delve deeper into the exploration of iron’s presence in nature, we unlock a greater understanding of the complex tapestry of existence and our responsibility to preserve its fragile intricacies.
Importance of Iron in Nature
In the vast ecosystem of our planet, the significance of iron cannot be overstated. This vital element plays a crucial role in myriad biological processes, functioning as a catalyst for various essential reactions. Iron acts as a catalyst by facilitating electron transfer, enabling organisms to carry out essential functions such as respiration, DNA synthesis, and photosynthesis. Furthermore, iron contributes to the structure and function of proteins, enabling them to undertake vital roles, such as oxygen transport and storage.
Iron’s ubiquitous presence in nature ensures its availability for an array of organisms and ecosystems. It is found in both terrestrial and aquatic environments, continually cycling through various biogeochemical processes. Whether it exists in the form of iron-rich minerals or dissolved in water, this versatile element serves as a critical nutrient for organisms ranging from microbes to plants and animals. Iron is vital for the growth and development of organisms, impacting their overall fitness and ecological interactions.
The ability of iron to form complexes with other molecules enhances its versatility and functionality in natural systems. These complexes enable iron to participate in redox reactions, transport electrons, and support enzymatic processes. Iron’s ability to switch between different oxidation states allows it to donate or accept electrons, facilitating energy metabolism and other essential physiological functions. Additionally, iron’s involvement in the creation of reactive oxygen species (ROS) underscores its crucial role in cellular signaling and defense mechanisms.
Despite its indispensability, iron’s environmental impact must also be considered. Human activities, such as industrial processes and the burning of fossil fuels, have led to elevated iron concentrations in ecosystems. This may disrupt the delicate balance of iron availability, affecting ecological processes and the health of organisms. It becomes imperative to mitigate anthropogenic iron inputs and ensure sustainable management practices to maintain and preserve the intricate iron-dependent dynamics in nature.
Iron in the Earth’s Crust
The presence of iron within the Earth’s crust is a phenomenon of great significance and ecological importance. It plays an essential role in various geological processes and has far-reaching impacts on the environment. This section explores the widespread occurrence of iron and its significance in shaping the Earth’s crust.
Iron, a metallic element, is abundantly present in the Earth’s crust, though its exact distribution varies across different regions. Its occurrence is not limited to a specific type of rock or mineral, as iron can be found in diverse geological formations. From sedimentary rocks to igneous formations and metamorphic rocks, iron is omnipresent, testament to its universal presence in the Earth’s outer layer.
The abundance of iron in the Earth’s crust is a key factor in its relevance to environmental processes. This element acts as a catalyst in numerous chemical reactions, leading to the formation and alteration of minerals and rocks. Iron-rich minerals, such as magnetite and hematite, contribute to the characteristic colors of various geological formations, adding to the scenic beauty of natural landscapes.
In addition, iron has profound ecological implications due to its role in supporting the growth and survival of organisms. It is an essential micronutrient for both plants and animals, playing a crucial role in important biochemical processes such as respiration and photosynthesis. Iron-rich soils provide a fertile environment for plant growth, enabling the sustenance of diverse ecosystems.
- Iron in various geological formations
- The abundance and distribution of iron
- Iron as a catalyst for chemical reactions
- Ecological importance of iron
Distribution of Iron Deposits
In this section, we will explore the distribution patterns of iron deposits found throughout various regions of the world. By examining these patterns, we can gain insight into the geographical diversity and occurrence of iron in nature.
Iron deposits can be found in numerous locations globally, exhibiting a wide range of characteristics and distributions. From vast reserves concealed beneath the Earth’s surface to smaller concentrations found in sedimentary deposits, the distribution of iron deposits is a testament to the diverse geological processes at work.
One notable distribution pattern of iron deposits is the clustering of high-grade ore deposits in specific regions. These regions, known for their abundant iron reserves, often become focal points for iron extraction and mining operations. Examples of such regions include the Pilbara region in Western Australia and the Brazilian Quadrilátero Ferrífero, both renowned for their extensive iron deposits.
Additionally, iron deposits can vary in their accessibility and form. Some iron deposits exist as concentrated ore bodies, easily exploitable through conventional mining techniques. Others, however, may be dispersed throughout rock formations or incorporated into complex mineral assemblages, posing challenges for extraction and processing.
Furthermore, the distribution of iron deposits is influenced by factors such as tectonic activity, weathering processes, and geological history. The formation of iron deposits often requires specific geological conditions, such as the presence of suitable iron-rich minerals, favorable temperature and pressure conditions, and the availability of fluid-rich environments for ore deposition.
Understanding the distribution of iron deposits is crucial for various reasons. It enables us to identify potential sources of iron, estimate global reserves, and assess the environmental impact of mining activities. By comprehending the intricate distribution patterns, we can make informed decisions regarding sustainable resource management and conservation efforts.
Types of iron ores
In this section, we will explore the various categories and classifications of iron ores found abundantly in different natural environments. Understanding the diversity of iron ores is essential in comprehending their environmental implications and impact.
Iron ores come in a variety of forms and compositions, each with its distinct properties and characteristics. These ores are a result of geological processes that have occurred over millions of years, leading to the formation of unique mineral deposits rich in iron.
One type of iron ore is hematite, which is known for its deep red color and high iron content. It often occurs in metamorphic and sedimentary rocks, and its fine-grained nature makes it an excellent source of iron for steel production.
Magnetite is another prominent type of iron ore, recognized for its magnetic properties and black color. This ore is often found in igneous and metamorphic rocks, and its magnetic nature makes it valuable in various industrial applications.
Another significant type of iron ore is taconite, characterized by its coarse-grained texture and lower iron content compared to hematite and magnetite. Taconite deposits are commonly found in sedimentary formations and are predominantly exploited for their iron content.
Other less common types of iron ores include limonite, goethite, and siderite. Limonite often occurs as a weathering product of other iron-bearing minerals and has a yellow-brown color. Goethite, on the other hand, can vary in color from yellow to brown and is commonly found in soils and sedimentary rocks. Siderite, with its pale yellow to brown color, is usually found in various types of carbonate deposits.
Each type of iron ore has its own unique properties and uses in different industries. By understanding the diversity of iron ores, we can better appreciate their abundance and environmental significance.
Extraction of Iron
In this section, we delve into the process of acquiring iron from natural sources, exploring various methods and techniques used to obtain this vital metal. Through a combination of scientific processes and technological advancements, iron extraction plays a significant role in meeting the ever-increasing demand for this essential element.
The extraction of iron involves a series of complex procedures that begin with the identification and selection of suitable iron-bearing ores. These ores are then subjected to various physical and chemical processes, including crushing, grinding, and magnetic separation, which aid in separating the iron-rich components from the unwanted impurities.
One common method utilized in the extraction of iron is the blast furnace process, which relies on the combustion of coke (a form of carbon) to facilitate the conversion of iron oxide into molten iron. This molten iron, known as pig iron, is then further refined through additional processes such as basic oxygen steelmaking or electric arc furnace to obtain the desired quality of iron for various industrial applications.
- The blast furnace process
- Alternative methods for iron extraction
- The role of coke in iron extraction
- Refining techniques for obtaining pure iron
By exploring the intricacies of iron extraction, we gain a deeper understanding of the immense efforts involved in harnessing this valuable resource, while also considering the environmental implications and sustainability practices associated with its extraction.
Iron Mining Techniques
In the realm of extracting iron from its natural sources, a wide array of techniques is employed to bring forth this vital metal. These methods leverage the abundance of iron in various natural formations and address the environmental implications of mining activities.
Firstly, one of the primary techniques used in iron mining is open-pit mining. This method involves the excavation of a large open pit or surface quarry, where iron ore deposits are located. The overlying soil and rocks are removed through blasting and drilling, allowing access to the iron ore underneath. Open-pit mining is often used for widespread iron ore deposits found near the surface.
Another commonly utilized technique for iron mining is underground mining. As the name suggests, this method involves the extraction of iron ore from underground mines. Shafts or tunnels are created to reach deeper iron ore deposits, and specialized machinery is used to extract the ore. Underground mining is employed when iron ore deposits are located at significant depths or when open-pit mining is not feasible.
Furthermore, a technique known as heap leaching is sometimes employed in iron mining. Heap leaching involves the processing of low-grade iron ore or crushed ore that cannot be directly smelted. In this technique, the ore is piled on a specially prepared pad and treated with chemicals to extract the iron. The leaching process allows for the separation and concentration of iron from the surrounding rock, making it economically viable to extract iron from lower-grade ores.
In addition to these primary mining techniques, various ancillary methods are used to optimize the extraction and processing of iron ores. These include blasting, crushing, grinding, and magnetic separation processes, which enhance the efficiency and purity of the extracted iron. Innovative technologies such as autonomous drilling and remote-controlled machinery are also increasingly utilized to improve safety and productivity in iron mining operations.
While iron mining techniques have greatly evolved over time, the quest for sustainability remains paramount. Efforts are made to minimize the environmental impact of mining activities, such as implementing stringent waste management practices, reclamation of mined areas, and the use of environmentally friendly mining equipment. These measures aim to ensure the responsible extraction and utilization of iron resources, securing the balance between meeting humanity’s iron needs and preserving the natural ecosystems.
Surface mining methods
Surface mining methods play a significant role in the extraction of valuable resources from the earth’s crust. These methods involve the removal of surface layers to access mineral deposits, including iron, found abundantly in nature. By utilizing a combination of innovative techniques, machinery, and safety protocols, surface mining operations have a considerable environmental impact.
Surface mining can be classified into several methods, each with its specific advantages and challenges. One commonly used method is open-pit mining, where large quantities of soil and rock are excavated to expose the underlying iron deposits. This method is highly efficient for extracting iron from near-surface deposits and allows for large-scale operations.
- Strip mining is another surface mining method that involves the removal of overburden, the layer above the iron deposit. This technique is particularly useful when the iron ore is located in layers parallel to the surface, making it easier to access and extract.
- Mountaintop removal mining is employed in areas where iron deposits are embedded within mountain ranges. In this method, the tops of mountains are blasted off, exposing the iron-rich layers underneath. While controversial due to its significant impact on the surrounding ecosystems, mountaintop removal mining can yield massive amounts of iron ore.
- Quarrying, often used for construction materials, can also be utilized for iron extraction. This method involves the cutting of large blocks or slabs of rocks, exposing the iron-bearing minerals within. Quarrying offers a precise and controlled way to extract iron from specific geological formations.
It is essential to consider the environmental implications of surface mining methods. Regardless of the specific technique employed, surface mining can cause deforestation, soil erosion, and habitat destruction. However, by implementing responsible mining practices, including land reclamation and minimizing the use of harmful chemicals, the environmental impact can be reduced.
In conclusion, surface mining methods are indispensable for accessing iron deposits abundantly available in nature. These techniques, such as open-pit mining, strip mining, mountaintop removal mining, and quarrying, offer effective ways to extract iron from various geological settings. However, it is crucial to balance the economic benefits with environmental stewardship to ensure the sustainable utilization of these valuable resources.
Underground mining methods
Exploration of subsurface extraction techniques
Delving deep into the Earth’s crust, underground mining methods unveil a wealth of possibilities for accessing precious resources. This section focuses on the techniques employed in extracting iron beneath the surface, away from the abundant layers of nature. By delving into the depths, miners venture into a parallel world, unearthing valuable ore deposits that have the potential to shape our modern society.
Diverse approaches to mining beneath the surface
When it comes to extracting iron via underground mining methods, there is no one-size-fits-all approach. Various techniques are utilized based on the geological characteristics of the deposit, ensuring the most efficient utilization of resources while minimizing environmental impact. These methods range from classical methodologies such as drift and slope mining to modern innovations like block caving and sublevel caving.
Utilizing the power of excavation
Within the realm of underground mining, excavation plays a vital role in uncovering the sought-after iron deposits. Tunnels, shafts, and drifts provide access to the ore body, allowing miners to reach the valuable resources hidden deep within the Earth. Specialized equipment, including drills, explosives, and loaders, are employed to carry out precise excavation operations, maximizing productivity while adhering to safety regulations.
Stability and safety considerations
The nature of underground mining poses unique challenges, requiring meticulous planning and execution to ensure the stability and safety of the operation. Engineers and geologists play a crucial role in assessing rock stability, implementing support systems, and monitoring potential hazards such as ground collapses or gas accumulation. By prioritizing safety measures and employing advanced monitoring techniques, miners can operate in a secure and responsible manner.
A symbiotic relationship with the environment
While underground mining methods are essential for accessing iron deposits hidden deep within the Earth, it is crucial to consider the environmental impact and strive for sustainable practices. Implementing progressive reclamation methods, monitoring of water and air quality, and reducing energy consumption are just a few approaches taken to minimize the ecological footprint of mining operations. By striking a balance between resource extraction and environmental preservation, we can ensure a harmonious coexistence for generations to come.
Iron in the Atmosphere
The Presence of Iron in the Sky
Iron, a ubiquitous element found abundantly in nature, is not only confined to the Earth’s surface. It also plays a significant role in the atmosphere, although it is often overlooked. This section aims to shed light on the intriguing presence of iron in the sky and explore its various implications and effects.
Iron particles can be found in the atmosphere as a result of natural processes such as dust storms, volcanic eruptions, and forest fires. These particles, varying in size and composition, have the potential to influence atmospheric phenomena and have a range of environmental impacts.
One of the key roles of iron in the atmosphere is its involvement in the formation and transformation of aerosols. Aerosols, tiny particles suspended in the air, have significant effects on climate and air quality. Iron particles can act as catalysts for the production of aerosols through chemical reactions, influencing their composition and size distribution.
Iron in Atmospheric Chemistry |
---|
Iron as a catalyst in aerosol formation |
Impact of iron particles on cloud formation |
Iron’s involvement in atmospheric deposition |
Iron in the atmosphere also interacts with other atmospheric components, such as oxygen and nitrogen compounds, contributing to the chemical reactions that occur in the sky. These reactions can lead to the production of different gases and compounds, some of which have important implications for human health and climate.
Furthermore, the transportation and deposition of iron particles in the atmosphere can have far-reaching consequences. Iron can be carried over long distances, spreading its influence across different regions. Upon deposition, iron can fertilize ecosystems such as oceans and affect the growth of phytoplankton, which in turn impact marine food webs and carbon cycling.
In conclusion, the presence of iron in the atmosphere goes beyond its role on Earth’s surface. Its interactions and effects in the sky are complex and diverse. Understanding the abundance and environmental impact of iron in the atmosphere is crucial for comprehending the intricate dynamics of our natural environment and its interconnections.
Iron Dust and Aerosols
In this section, we will explore the presence and effects of iron dust and aerosols in various natural environments. We will delve into the diverse forms and sources of these iron particles, as well as their dispersion and transport mechanisms. Additionally, we will examine the potential ecological and atmospheric impacts associated with iron dust and aerosols.
Iron dust and aerosols, commonly found in nature, play a significant role in numerous environmental processes. These tiny particles, ranging in size from fine dust to microscopic aerosols, originate from a multitude of natural sources such as volcanic eruptions, soil erosion, and meteorological events. They can also arise from anthropogenic activities, including industrial emissions and combustion processes.
The dispersal of iron particles occurs through various mechanisms, including wind and water currents. This allows them to travel across vast distances, affecting ecosystems and atmospheric conditions far from their original source. Additionally, the size and composition of iron dust and aerosols can influence their behavior in the environment, with certain particles having the potential to serve as carriers for other substances or act as catalysts in chemical reactions.
- Volcanic eruptions: Volcanoes release significant amounts of iron-rich dust and aerosols during explosive eruptions. These particles can be transported thousands of kilometers, with the potential to impact climate patterns and contribute to nutrient enrichment in marine ecosystems.
- Desert dust storms: Arid regions, such as deserts, generate substantial amounts of iron dust through wind erosion. These dust storms can distribute iron particles over long distances, affecting air quality and contributing to the deposition of essential nutrients onto terrestrial and aquatic ecosystems.
- Industrial emissions: Various industrial processes release iron-containing particulate matter into the atmosphere. These emissions can have detrimental effects on air quality and human health, highlighting the need for effective pollution control measures.
Understanding the abundance and distribution of iron dust and aerosols is crucial for comprehending their environmental impacts. The deposition of iron particles can influence biogeochemical cycles, affecting nutrient availability and productivity in both terrestrial and aquatic ecosystems. Additionally, interactions between iron particles and atmospheric gases can contribute to the formation of pollutants and impact climate dynamics.
By exploring the characteristics and consequences of iron dust and aerosols, we can gain insights into their vital role in shaping Earth’s ecosystems and climatic conditions. This knowledge is essential for developing sustainable strategies that mitigate the potential environmental impacts associated with the presence of iron in nature.
Sources and transportation
In this section, we will delve into the origins and movement of iron in the natural environment. Understanding the various sources and the mechanisms by which iron is transported is essential for comprehending its abundance and environmental implications.
Iron can be found in a multitude of sources throughout the Earth’s crust, ranging from volcanic activity to sedimentary rock formations. These sources contribute to the overall availability of iron in nature, making it a vital element in numerous ecological processes.
One significant source of iron is through weathering processes, where external factors such as rainfall, temperature variations, and chemical interactions gradually break down iron-containing minerals. This process releases iron into the surrounding environment and contributes to its dispersion. Additionally, iron-rich deposits can also be found in various geological formations, such as banded iron formations, which have played a crucial role in Earth’s history.
The transportation of iron occurs through a variety of natural channels, including the hydrosphere, atmosphere, and biosphere. In aquatic environments, iron can be transported through water bodies and rivers, either as dissolved ions or bound to particles and sediments. Atmospheric transportation involves iron being carried by winds and deposited in distant locations, contributing to the global distribution of this element. Biological processes, such as the uptake of iron by plants and the subsequent movement through food chains, also play a role in the transfer of iron within ecosystems.
Understanding the intricate network of sources and transportation pathways is essential for unraveling the complex dynamics of iron in nature. By comprehending these processes, we can gain insights into the environmental impact of iron and make informed decisions regarding its management and conservation.
Iron in Water Bodies
The presence of iron in water bodies plays a significant role in shaping their ecosystems. This element, known for its distinct properties, has a profound influence on the aquatic environment. Iron concentrations in water bodies can vary greatly, affecting the overall chemistry and biological functioning of these ecosystems. Understanding the abundance and behavior of iron in different water bodies is crucial for comprehending its environmental impact and potential implications for organisms within these aquatic systems.
Iron in Freshwater | Iron in Marine Environments |
---|---|
Freshwater bodies, such as rivers, lakes, and ponds, contain varying levels of iron. This element can originate from natural sources, such as weathering of rocks and soils, as well as from human activities, including industrial discharges and agriculture runoff. | In marine environments, the presence of iron is critical for numerous marine organisms. It serves as an essential micronutrient for phytoplankton, which are the foundation of marine food webs. Iron availability influences primary productivity and can impact the overall structure and dynamics of marine ecosystems. |
Iron in water bodies can exist in different forms, including soluble ferrous (Fe2+) and insoluble ferric (Fe3+) ions. The solubility of iron is influenced by various factors, such as pH, oxygen levels, and the presence of organic matter. These factors contribute to the cycling of iron in aquatic systems, affecting its availability for biological uptake and precipitation as iron-containing minerals.
The effects of iron on aquatic organisms can be both beneficial and detrimental. Iron plays a crucial role in biological processes, such as oxygen transport, enzymatic reactions, and cell growth. However, high concentrations of iron can lead to toxicity, impairing the growth and survival of aquatic species. The complex interactions between iron and other elements in water bodies warrant further investigation to fully understand its ecological implications.
Overall, the study of iron in water bodies is vital for comprehending the intricate relationship between this element and the environment. The abundance, behavior, and impacts of iron in freshwater and marine ecosystems have significant implications for the functioning and sustainability of these natural systems. By examining the role of iron in different water bodies, scientists can enhance their understanding of the overall biogeochemical cycles and ecological dynamics in aquatic environments.
Iron Concentration in Rivers
Rivers are important sources of freshwater, serving as lifelines for both humans and ecosystems. Within these flowing bodies of water, there exists a significant presence of iron, a vital element with diverse ecological implications. This section aims to delve into the various factors influencing iron concentration in rivers and explore its ecological importance.
Iron, an essential micronutrient, is naturally present in the Earth’s crust and is commonly found in various forms, including dissolved, particulate, and colloidal forms. The concentration of iron in rivers is influenced by a multitude of factors such as geological processes, weathering of rocks and minerals, precipitation patterns, and human activities. Understanding these factors is crucial to comprehending the distribution and abundance of iron in river systems.
- Geological Processes: The geological composition of a river’s catchment area plays a significant role in determining the iron concentration in the water. Different types of rocks and soil have variable iron content, which impacts the amount of iron released into the river through weathering and erosion processes.
- Weathering and Erosion: The physical breakdown of rocks and minerals through weathering and erosion releases iron particles into the river. Climate conditions, including temperature, precipitation, and wind patterns, influence the rate of weathering, thereby affecting the iron concentration in rivers.
- Precipitation Patterns: Rainfall patterns, as well as seasonal variations, can impact the iron concentration in rivers. Heavy rainfall events can cause runoff, carrying sediment and dissolved iron from the land into the river, while dry periods may reduce the inflow of iron-bearing materials.
- Human Activities: Human activities such as agriculture, mining, and industrial processes can significantly alter iron concentrations in rivers. Runoff from fertilizers, mining activities, and untreated industrial waste can introduce high levels of iron into river systems, leading to potential environmental impacts.
The ecological importance of iron in rivers cannot be overstated. Iron plays a crucial role in various biogeochemical and ecological processes, including nutrient cycling, primary production, and oxygenation of aquatic systems. Furthermore, iron availability can influence the growth and development of aquatic organisms, including phytoplankton, zooplankton, and fish, thus impacting the overall biodiversity and ecosystem functioning.
In conclusion, the concentration of iron in rivers is influenced by geological processes, weathering, precipitation patterns, and human activities. Understanding these factors and their ecological implications is crucial for assessing the overall health and functioning of river ecosystems.
Influencing factors
The abundance and impact of iron in the natural environment are determined by a multitude of factors that shape its presence and behavior. By exploring the various influencing factors, we can gain deeper insights into the complex dynamics of iron in nature.
One significant influencing factor is the geographical location. Iron distribution and availability vary across different regions, influenced by factors such as climate, geological formations, and soil composition. These factors can affect the abundance of iron in terrestrial ecosystems, including forests, grasslands, and wetlands.
Another key factor is the presence of other elements and compounds in the environment. The interactions between iron and other substances, such as oxygen, carbon, and sulfur, can greatly influence its chemical forms and reactivity. Understanding these interactions is crucial for comprehending the environmental impact of iron and its role in various natural processes.
The availability of iron also depends on specific environmental conditions, such as pH and redox potential. Iron solubility and mobility are influenced by the acidity or alkalinity of the surroundings, as well as the presence of oxidizing or reducing agents. These factors can affect the bioavailability of iron to organisms and its participation in biogeochemical cycles.
Furthermore, human activities can significantly influence the abundance and environmental impact of iron. Industrial processes, mining operations, and pollution contribute to the alteration of iron levels in natural systems and may lead to detrimental effects on ecosystems. Understanding the anthropogenic factors at play is crucial for proposing sustainable solutions and mitigating the potential negative consequences of iron in the environment.
Influencing Factors | Description |
---|---|
Geographical Location | Climate, geology, and soil composition affecting iron distribution |
Presence of Other Elements | Interactions with oxygen, carbon, sulfur, and other substances shaping iron’s behavior |
Environmental Conditions | pH, redox potential, and their impact on iron solubility and mobility |
Human Activities | Industrial processes, mining, and pollution altering iron levels |
Ecological Consequences
Within the realm of natural ecosystems, the presence of iron exerts significant ecological consequences that extend far beyond a simple exploration of its abundance and environmental impact in nature. The intricate interplay between iron and various biological processes plays a fundamental role in shaping the delicate balance of ecosystems.
Iron, being an essential nutrient for all forms of life, influences the growth and development of organisms across the ecological spectrum. It is involved in crucial physiological processes such as oxygen transport, energy production, and enzymatic reactions. The availability or scarcity of iron in an ecosystem can directly affect the population dynamics and distribution of organisms, ultimately cascading up or down the food chain.
In aquatic environments, iron plays a fundamental role in the growth of phytoplankton, microscopic photosynthetic organisms that form the basis of the aquatic food web. Phytoplankton productivity heavily relies on iron availability, with low iron concentrations often limiting their growth potential. This limitation can have far-reaching consequences for higher trophic levels, such as zooplankton and fish, which depend on phytoplankton as a primary food source.
Furthermore, the ecological consequences of iron extend to the cycling of other elements within ecosystems. Iron can act as a catalyst for various chemical reactions that are key in nutrient cycling, such as nitrogen fixation and the oxidation of organic matter. The availability of iron can influence the rates at which these essential processes occur, ultimately impacting nutrient availability and the overall productivity of ecosystems.
Iron’s role in the regulation of greenhouse gases
Implications for biodiversity and ecosystem resilience
The influence of iron on soil health and nutrient cycling
Iron’s impact on the stability of aquatic ecosystems
Effects of iron deficiency or excess on plant growth and productivity
In conclusion, the ecological consequences of iron in nature are multifaceted and profound. Its availability shapes the intricate web of life, influencing the growth and distribution of organisms, nutrient cycling, and ecosystem productivity. Understanding the ecological consequences of iron is pivotal for effective conservation and sustainable management of natural resources.
Impact of Iron on Marine Life
Influence of Ferrous Compound on Aquatic Organisms
The presence of iron in marine environments has a significant influence on the biodiversity and overall health of marine life. Iron plays a crucial role in various biological processes, affecting both plants and animals that inhabit oceans and seas.
Iron availability affects the growth and development of phytoplankton, which are vital primary producers in marine ecosystems. Phytoplankton utilize iron for photosynthesis, and the limitation of this nutrient can limit their growth, leading to potential changes in the entire food chain of marine organisms. Furthermore, iron deficiency can negatively affect the metabolic processes and reproduction of marine plants, impacting the availability of food and habitat for other marine species.
Iron also affects the physiology and behavior of marine animals, such as fish and crustaceans. Iron deficiency can lead to reduced oxygen transportation in the bloodstream, affecting the overall fitness and survival of these organisms. Additionally, iron accumulation in certain marine species can have toxic effects, leading to impaired growth, reproduction, and increased susceptibility to diseases.
- Iron is involved in regulating the gene expression and enzymatic activity in marine organisms, affecting their overall response to environmental stressors.
- The presence of iron in marine sediments can impact the composition and structure of benthic communities, as certain organisms are more sensitive to iron concentrations than others.
- Iron also plays a role in the carbon and nitrogen cycling in marine ecosystems, influencing nutrient availability and productivity.
Overall, understanding the impact of iron on marine life is essential for the management and conservation of marine ecosystems. Ensuring proper iron levels and availability in marine environments is crucial for maintaining biodiversity and the sustainability of marine ecosystems.
Iron Fertilization
The concept of enhancing biological productivity in the natural environment through the addition of iron presents a fascinating perspective on the utilization and potential consequences of this trace metal. This approach, known as iron fertilization, aims to stimulate phytoplankton growth in nutrient-poor regions by introducing iron compounds into marine ecosystems. The effects of this intervention have been of great interest to scientists and environmentalists, as they seek to understand the implications and benefits of such an action.
Iron fertilization holds promise as a means to enhance carbon sequestration and mitigate the effects of climate change. When iron is added to oceanic waters, it acts as a catalyst for the growth of phytoplankton, a cornerstone of oceanic food webs. Increased phytoplankton populations lead to higher carbon dioxide absorption, helping to reduce greenhouse gas concentrations in the atmosphere. Additionally, the resulting increase in marine productivity can have far-reaching impacts, supporting fisheries and providing habitats for various species.
However, the careful consideration of potential drawbacks and unintended consequences is crucial when contemplating widespread implementation of iron fertilization projects. While it may boost biological productivity in certain regions, it is essential to understand the specific circumstances under which iron fertilization can be effective and sustainable. Excessive iron addition can lead to the overgrowth of certain species, potentially causing harmful algal blooms or disrupting the natural balance of marine ecosystems.
Research efforts are ongoing to determine the most suitable conditions for successful iron fertilization, including the choice of iron compounds, dosage, and timing of application. Scientists recognize the need for an interdisciplinary approach, considering the complex interactions between iron availability, nutrient levels, and ecological dynamics. Evaluating the long-term effects of iron fertilization requires careful monitoring and assessment, taking into account not only the immediate changes but also the potential cascading impacts on different trophic levels.
In conclusion, iron fertilization offers a captivating avenue for exploring the potential benefits and challenges associated with manipulating the iron content in marine environments. While it holds promise as a tool for carbon sequestration and ecosystem enhancement, extensive research and precautionary measures are necessary to ensure the sustainability and minimize unintended consequences of this intervention.
Effects on primary productivity
Impacts on the growth and development of primary producers can be observed as a consequence of the presence of iron in various natural systems. These effects on primary productivity encompass a wide range of ecological processes and can shape the overall functioning of ecosystems.
The influence of iron on primary productivity is multifaceted, with both direct and indirect effects. Iron availability can directly affect the physiological processes of primary producers, such as photosynthesis and nutrient uptake, influencing their growth rates and biomass accumulation. Additionally, iron can indirectly impact primary productivity by altering the composition of the microbial community associated with primary producers, leading to changes in nutrient cycling and availability.
Furthermore, variations in iron concentrations can result in shifts in species composition and dominance within primary producer communities. Some species may show increased growth and competitive advantage under iron-enriched conditions, while others may be hindered in their development, leading to alterations in species diversity and community structure.
The effects of iron on primary productivity have significant implications for ecosystem functioning and stability. Changes in primary productivity can cascade through the food web, impacting higher trophic levels and overall ecosystem dynamics. Additionally, alterations in primary productivity can affect carbon sequestration and nutrient cycling, with potential consequences for global biogeochemical cycles.
In conclusion, the effects of iron on primary productivity are integral to understanding the ecological functioning of natural systems. By investigating the various ecological processes influenced by iron availability, we can gain insights into the intricate interactions between biotic and abiotic factors and their impact on ecosystem dynamics.
Iron in Agricultural Soils
Agricultural soils play a crucial role in the overall availability and distribution of iron in nature. This section focuses on exploring the presence of iron in soils specifically used for agriculture, highlighting its significance, diverse forms, and potential environmental implications.
Iron, an essential micronutrient for plants and crops, is found abundantly in agricultural soils. Its presence in these soils greatly influences the growth and development of various agricultural produce. Iron exists in different forms, including oxidized and reduced states, which impact its availability for uptake by plants. The dynamics of iron in agricultural soils vary depending on environmental factors, such as pH levels, organic matter content, and soil erosion, among others.
Agricultural practices and management techniques significantly impact the concentration and availability of iron in soils. The use of fertilizers, irrigation methods, and crop rotations can either enhance or deplete iron content. Understanding the interactions between iron and agricultural systems is crucial for optimizing crop production while mitigating potential environmental impacts.
- Iron Deficiency in Agricultural Soils: In certain agricultural regions, iron deficiency can limit crop productivity and quality. This issue arises due to factors like alkaline soils, high pH levels, and inadequate iron availability. Implementing suitable soil amendments and efficient agronomic practices can help address these challenges and improve iron availability for plants.
- Iron Toxicity in Agricultural Soils: Excessive iron concentrations in soils can lead to toxicity, adversely affecting plant growth and productivity. Factors such as improper drainage, acidification, and excessive iron application contribute to iron toxicity. Balancing nutrient management and adopting suitable soil remediation techniques are essential for minimizing the negative impacts of iron toxicity in agricultural soils.
- Environmental Implications: The management of iron in agricultural soils also has implications for the surrounding environment. Excessive iron runoff from agricultural lands can contribute to water pollution, negatively impacting aquatic ecosystems. Additionally, the emissions of greenhouse gases from soil perturbations during agricultural practices should be considered in order to minimize environmental harm.
In conclusion, understanding the presence and dynamics of iron in agricultural soils is vital for sustainable and productive farming. Efficient management practices can enhance iron availability for plants, while also mitigating potential environmental risks associated with its presence. A balanced approach that takes into account the specific soil conditions and agricultural requirements is essential for optimizing iron utilization in agricultural systems.
Iron Deficiency in Plants
Insufficient iron levels can have a detrimental effect on the growth and development of plants. This depletion of iron in plants, also referred to as iron deficiency, can hinder various metabolic processes essential for their survival.
Impact on Plant Health: When plants lack adequate iron, they experience a range of symptoms that can compromise their overall health and vigor. Leaves may exhibit yellowing, also known as chlorosis, due to reduced chlorophyll production. This leads to decreased photosynthesis, impeding the plants’ ability to generate energy and vital nutrients.
Role of Iron in Plants: Iron is crucial for numerous cellular functions in plants. It plays a significant role in the synthesis of chlorophyll, which is essential for photosynthesis. Moreover, iron facilitates the transport of electrons, facilitating electron transport chains and energy production. It is also involved in enzyme activation, DNA synthesis, and nitrogen fixation.
Causes of Iron Deficiency: Iron deficiency in plants can arise from multiple factors. Soil pH significantly affects iron availability, as excessive alkalinity or acidity can limit the uptake of iron by plant roots. Additionally, excessive phosphorus levels, high soil moisture, and the presence of other minerals, such as manganese or zinc, can interfere with iron absorption.
Addressing Iron Deficiency: Various approaches can be employed to combat iron deficiency in plants. Soil amendments, including the application of iron chelates or organic matter, can enhance iron availability. Adjusting soil pH through the addition of appropriate amendments can also improve iron uptake. Furthermore, enhancing root health through proper irrigation and drainage practices can facilitate efficient iron uptake and utilization.
Conclusion: Recognizing the significance of iron for plant growth and understanding the causes and symptoms of iron deficiency are crucial for ensuring optimal plant health and productivity. By implementing appropriate strategies to address iron deficiency, the negative impacts on plant growth can be minimized, thereby promoting a healthier and more resilient plant ecosystem.
Signs and symptoms
The manifestations and indications associated with the presence and effects of iron in the natural environment are varied and multifaceted. Recognizing these signs and symptoms is pivotal in comprehending the impact of this element on our surroundings. By understanding the diverse ways in which iron manifests itself, we can delve deeper into its role and consequences in nature.
Below are some of the prevalent signs and symptoms that can be observed when iron is present in abundance:
- Elevated levels of iron can lead to a distinct alteration in the coloration of various natural environments, ranging from vivid reds and oranges to more subtle shades of brown.
- The excessive accumulation of iron can result in detrimental effects on flora and fauna. It can disrupt the growth and survival of plants and significantly impact the composition and diversity of aquatic ecosystems.
- Iron-rich environments often exhibit peculiar smells, characterized by a metallic or earthy odor that can be discernible even from a distance.
- Exposure to excessive iron levels can lead to corrosion of infrastructure and equipment, posing economic challenges in industries and areas where iron plays a vital role.
While these signs and symptoms provide a glimpse into the presence and impact of iron in nature, it is crucial to delve deeper into each specific context to fully comprehend its environmental significance. Acknowledging these indicators allows us to better understand the complexities of iron abundance and its repercussions on the natural world.
Treatment and prevention
Tackling the Management and Mitigation of Iron-related Issues
Addressing challenges related to iron abundance and its environmental implications demands a comprehensive understanding of treatment and prevention strategies. This section comprehensively explores various measures and approaches to manage and mitigate iron-related problems, aiming to safeguard the delicate balance of nature.
Preventing excess iron accumulation is crucial in maintaining ecosystem health.
1. Iron Chelation Therapy:
One approach in the treatment of iron overload disorders involves iron chelation therapy, which aims to reduce the excess iron in the body. Iron chelators, such as deferoxamine and deferiprone, form stable complexes with iron ions, facilitating their excretion from the body. This therapeutic strategy has shown promising results in treating conditions like hemochromatosis, where iron accumulates to toxic levels.
2. Biological Processes:
Nature itself offers potential solutions for mitigating iron-related environmental impact. Microorganisms play a significant role in biogeochemical iron cycles, participating in a range of processes like iron oxidation and reduction. Understanding these natural processes can help develop bioremediation strategies to restore contaminated ecosystems.
3. Water Treatment Technologies:
Efficient water treatment technologies are crucial to remove excess iron from drinking water sources, protecting human health and infrastructure. Processes such as chemical precipitation, filtration, and oxidation are commonly employed to reduce iron concentrations to acceptable levels. Developing innovative technologies and improving existing methods are vital to ensure clean water supplies and prevent iron-related health risks.
4. Environmental Monitoring:
Rigorous monitoring programs are essential in identifying areas at risk of iron accumulation, both in natural and industrial environments. Various analytical techniques, such as atomic absorption spectrometry and inductively coupled plasma mass spectrometry, aid in accurately assessing iron concentrations. Timely detection and comprehensive monitoring help to inform preventive measures and minimize the potential ecological impact.
In summary, effective treatment and prevention strategies are indispensable in tackling the challenges associated with iron abundance in nature. By employing iron chelation therapy, harnessing biological processes, implementing water treatment technologies, and conducting thorough environmental monitoring, we can work towards maintaining a harmonious coexistence with iron in the natural world.