Understanding the Physics Behind the Science of Microwave Heating
Microwave heating: principles, applications, and safety considerations for efficient and uniform heating of materials.
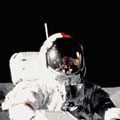
- Anthony Arphan
- 31 min read
Microwave ovens have become an indispensable tool in today’s fast-paced kitchen. From heating up leftovers to cooking a full meal from scratch, these appliances offer convenience and speed. But have you ever wondered how microwaves actually work? Understanding the physics behind microwave heating can help us appreciate the technology and make the most of our microwave ovens.
At the heart of microwave heating is a phenomenon known as dielectric heating. Dielectric materials, such as food, have the ability to absorb and convert electromagnetic waves into heat energy. In a microwave oven, this process is achieved through the generation of microwave radiation, which is made up of high-frequency electromagnetic waves.
When the microwave oven is turned on, electromagnetic waves are produced by a component called the magnetron. These waves are then directed into the cooking chamber, where they interact with the food. The microwaves penetrate the food and cause the water molecules within it to vibrate at a rapid rate. This vibration generates friction and heat, resulting in the food’s temperature rising.
It is worth noting that microwave ovens mainly heat water molecules due to their unique structure. Since water molecules are polar, meaning they have positive and negative charges, they rapidly rotate as they try to align themselves with the changing electromagnetic field. This rotation generates heat and explains why some foods, particularly those with higher water content, heat more quickly in the microwave.
In conclusion, the science behind microwave heating relies on dielectric heating, which involves the absorption of microwave radiation by food. The generation of high-frequency electromagnetic waves causes water molecules within the food to vibrate and generate heat. This understanding of the physics behind microwave heating can help us make informed decisions about cooking times and power levels when using these convenient kitchen appliances.
The Physics Behind Microwave Heating
Microwave ovens work by using electromagnetic waves to heat food. These waves are produced by an electronic device called a magnetron, which converts electricity into high-frequency radio waves. These radio waves are then directed into the cooking chamber of the microwave, where they interact with the food.
The key to understanding microwave heating lies in the properties of the waves themselves. Microwave radiation has a unique ability to penetrate deep into the interior of food, whereas conventional heat sources like ovens or stovetops can only heat the surface. This is due to the fact that microwaves have a longer wavelength than visible light or infrared radiation, allowing them to pass through materials like plastic or glass.
When the microwaves come into contact with the food, they are absorbed by water molecules present in the food. This is because microwaves cause the water molecules to oscillate rapidly, a process known as dielectric heating. The rapid movement of the water molecules generates heat, which is then transferred to the surrounding food.
This unique heating mechanism offers several advantages over traditional cooking methods. Firstly, microwave heating is much faster than conventional methods, as the waves can penetrate the food and heat it evenly from the inside out. Secondly, it is more energy-efficient, as microwave ovens only heat the food itself and not the surrounding air or cookware. Finally, microwave heating can preserve the nutrients in food better than other cooking methods, as the shorter cooking times minimize the loss of heat-sensitive vitamins and minerals.
In conclusion, the physics behind microwave heating is a fascinating topic that explains how electromagnetic waves can be used to rapidly and efficiently heat food. By understanding the unique properties of microwave radiation, we can appreciate the many benefits that microwave ovens offer in terms of speed, energy efficiency, and nutrient preservation.
Electromagnetic Waves and Microwaves
The science behind microwave heating lies in understanding electromagnetic waves and how they interact with matter. Electromagnetic waves are a form of energy that can travel through space in the absence of any medium. They are characterized by their wavelength and frequency, which are inversely related.
Microwaves are a specific type of electromagnetic wave that falls within the spectrum of radio waves. They have a wavelength ranging from about one millimeter to one meter and a frequency of around 300 gigahertz to 300 megahertz. Microwaves are used in many applications, including communication, radar, and of course, heating food.
When microwaves interact with matter, such as food, they can be absorbed, transmitted, or reflected. The absorption of microwaves by the food is what leads to the heating effect. At the molecular level, microwaves cause water molecules to rapidly oscillate, generating heat in the process. This is why microwaves are particularly effective at heating water-based substances.
Furthermore, microwaves have the ability to penetrate deeper into food compared to other heating methods, such as conduction or convection. This is because microwaves can easily pass through materials like glass, paper, and plastic, allowing for a more even and efficient heating process.
In summary, microwaves are a type of electromagnetic wave that can be absorbed by matter, leading to a heating effect. Their ability to penetrate through materials and their specific interaction with water molecules make them an efficient and convenient method for heating food.
Understanding Electromagnetic Spectrum
The electromagnetic spectrum is a range of electromagnetic radiation that covers a wide range of wavelengths and frequencies. It includes everything from radio waves and microwaves to infrared, visible light, ultraviolet, X-rays, and gamma rays.
Each type of electromagnetic radiation has a unique wavelength and frequency, which determines its properties and how it interacts with matter. Radio waves, for example, have long wavelengths and low frequencies, while gamma rays have short wavelengths and high frequencies.
Understanding the electromagnetic spectrum is crucial in microwave heating because microwaves are a type of electromagnetic radiation. Microwaves have longer wavelengths and lower frequencies compared to visible light, making them ideal for heating food and other substances.
When an object is placed in a microwave oven, the microwaves penetrate the item and interact with the water molecules inside. The microwaves excite the water molecules, causing them to vibrate and generate heat. This process is known as dielectric heating and is the main mechanism behind microwave heating.
By understanding the properties of different types of electromagnetic radiation and how they interact with matter, scientists can design microwave ovens and other technologies that harness this energy for various applications. The study of the electromagnetic spectrum continues to reveal new insights into the physics of microwave heating and its potential uses in fields like food processing, material science, and medical research.
Characteristics of Microwaves
Microwaves are a form of electromagnetic radiation with wavelengths ranging from about 1 millimeter to 1 meter. They are classified as a type of radio wave and can be found on the electromagnetic spectrum between infrared radiation and radio waves.
Here are some key characteristics of microwaves:
- Wavelength: Microwaves have relatively long wavelengths compared to other types of electromagnetic radiation. This allows them to pass through many materials that would block or reflect shorter waves, such as visible light.
- Penetration: Microwaves have the ability to penetrate and interact with certain materials, such as food and liquids. This is why they are commonly used in microwave ovens to heat and cook food.
- Heating: Microwaves can cause substances to heat up by exciting the molecules within them. This is due to the interaction between the microwaves and the polar molecules present in the material.
- Directivity: Microwaves can be directed and focused using various techniques, such as reflectors and antennas. This allows for targeted heating or communication over long distances.
- Speed: Like all forms of electromagnetic radiation, microwaves travel at the speed of light in a vacuum, which is about 299,792 kilometers per second.
- Frequency: Microwaves have frequencies ranging from about 300 megahertz (MHz) to 300 gigahertz (GHz). This high frequency range allows for efficient transmission and reception of microwaves.
Understanding the characteristics of microwaves is crucial in various fields, from microwave technology and communication to the design and operation of microwave cooking appliances.
Interaction of Microwaves with Molecules
Microwaves, a form of electromagnetic radiation, interact with molecules through a process known as dielectric heating. This interaction occurs when the electric field of the microwave radiation causes charged particles within the molecule to oscillate. These charged particles include electrons, protons, and other polar molecules.
When a microwave field interacts with a molecule, it exerts a force on the charged particles within the molecule, causing them to move back and forth. This movement generates heat through friction, resulting in the overall increase in temperature of the molecule.
The key factor in the interaction between microwaves and molecules is the molecule’s dipole moment, which measures the separation of positive and negative charges within the molecule. Molecules with higher dipole moments tend to experience stronger interaction with microwaves and are more efficient at converting microwave energy into heat.
Furthermore, the specific frequency of the microwave radiation influences the interaction with the molecules. The molecules resonate with certain frequencies, and when the frequency of the microwave radiation matches the resonant frequency of the molecule, a more efficient energy transfer occurs. This phenomenon is known as resonance absorption.
The interaction of microwaves with molecules has various practical applications. In microwave ovens, for example, water molecules in food are particularly effective at absorbing microwave radiation due to their high dipole moment. This absorption leads to the heating of the food. Additionally, microwave technology is used in chemical synthesis, where targeted heating of specific molecules can accelerate reactions and improve yields.
In summary, the interaction of microwaves with molecules involves the oscillation of charged particles within the molecule, generating heat through friction. The dipole moment and resonance frequency of the molecule play crucial roles in the efficiency of this interaction. Understanding these factors helps to unlock the potential of microwave technology in various fields.
Dielectric Heating Mechanism
The dielectric heating mechanism is the primary process by which microwaves generate heat in materials. It relies on the interaction between the electromagnetic fields of the microwave radiation and the electric dipoles within the material. Electric dipoles are molecules or atoms that have a positive and negative charge separated by a small distance.
When a material is exposed to microwaves, the electric fields cause the electric dipoles to align with the alternating field. As the field rapidly changes direction, the dipoles try to reorient themselves, leading to molecular movement and heat generation. This movement results in the frictional heating of the material.
The efficiency of the dielectric heating mechanism depends on the dipolar properties of the material. Materials with high dipole moments, such as water and many organic compounds, are particularly effective at absorbing microwave radiation and converting it into heat. In contrast, materials with low dipole moments, such as metals and ceramics, are generally poor absorbers of microwaves.
One key advantage of dielectric heating is its selective heating ability. Since different materials have different dipolar properties, microwaves can be used to selectively heat specific substances in a mixture. For example, if a mixture contains both water and oil, the microwaves will mostly heat the water due to its higher dipole moment, while leaving the oil relatively unaffected.
In addition to the selective heating, dielectric heating also offers rapid and uniform heating. The penetration depth of microwaves is typically a few centimeters, allowing for efficient heating throughout the material. The rapid and uniform heating can be especially advantageous in industrial processes where quick and even heating is required.
The dielectric heating mechanism plays a crucial role in various applications of microwaves, including cooking, chemical synthesis, and material processing. Understanding the physics behind this process is essential for harnessing the full potential of microwave heating technology.
Molecular Rotations and Vibrations
When microwaves interact with molecules, they can cause rotations and vibrations within the molecular structure. These rotations and vibrations play a crucial role in determining the heating properties of microwaves and the overall efficiency of microwave heating processes.
Molecular rotations occur when molecules absorb microwave radiation and start to rotate around their centers of mass. This rotational motion leads to friction and collisions between neighboring molecules, generating heat. The ability of a molecule to rotate depends on its size, shape, and overall symmetry. For example, small molecules with low moments of inertia can rotate easily, while larger and more complex molecules may have restricted rotation due to their size and shape.
Molecular vibrations, on the other hand, involve the stretching and bending of chemical bonds within a molecule. When microwaves interact with a molecule, they can excite these vibrational modes, causing the bonds to stretch and compress. Similar to molecular rotations, these vibrations generate heat through friction and collisions between neighboring molecules.
The efficiency of microwave heating is influenced by the ability of microwaves to match the resonant frequencies of molecular rotations and vibrations. By adjusting the frequency and power of the microwave radiation, it is possible to selectively target specific molecular bonds and enhance their rotations and vibrations. This selective heating can be used to achieve precise temperature control and speed up chemical reactions in various industrial processes.
Microwave Heating Process | Molecular Rotations and Vibrations |
---|---|
Food Preparation | Microwaves cause rotations and vibrations in water molecules, generating heat and cooking the food. |
Chemical Synthesis | Microwaves selectively excite specific molecular bonds, enhancing rotations and vibrations to accelerate chemical reactions. |
Materials Processing | Microwaves induce rotations and vibrations in certain materials, allowing for controlled heating and shaping. |
Understanding the molecular rotations and vibrations induced by microwaves is crucial for optimizing microwave heating processes and exploring new applications in various fields. Researchers continue to investigate the physics behind these phenomena, seeking to unravel the intricacies of microwave-matter interactions.
Penetration Depth and Absorption
Penetration depth and absorption are fundamental concepts in understanding the science behind microwave heating. When microwaves interact with a material, they penetrate into the material and are absorbed by it. The penetration depth is the distance that the microwaves can travel into the material before being significantly attenuated or absorbed.
The penetration depth depends on the frequency of the microwaves and the properties of the material. At higher frequencies, the penetration depth is smaller. This is why microwave ovens typically operate at 2.45 GHz, as this frequency is well-suited for heating food without penetrating too deeply into the food.
The absorption of microwaves by a material is determined by its dielectric properties. Dielectric properties describe the response of a material to an electric field. Materials with high dielectric constants absorb microwaves more effectively than materials with low dielectric constants.
Water is an example of a material with high dielectric constant, which is why it absorbs microwaves so effectively. This is why food, which contains a lot of water, heats up quickly in a microwave oven. On the other hand, materials with low dielectric constants, such as certain plastics and glass, absorb microwaves poorly and instead reflect or transmit them.
Understanding the penetration depth and absorption of microwaves is crucial in designing microwave heating systems. By optimizing the frequency and choosing materials with appropriate dielectric properties, it is possible to efficiently heat and cook various materials using microwaves.
Skin Effect and Loss Tangent
Microwave heating is a process in which electromagnetic waves are used to heat materials through the interaction of the waves with the molecules in the material. One important phenomenon that occurs during microwave heating is the skin effect. The skin effect is the tendency of the electromagnetic field to be more concentrated near the surface of the material, causing it to heat up more quickly.
The skin effect is caused by the high frequency of the electromagnetic waves used in microwave heating. At higher frequencies, the electromagnetic field has a shorter wavelength, which leads to a more concentrated field near the surface of the material. This concentrated field causes the molecules near the surface to vibrate more rapidly, generating heat.
The depth at which the electromagnetic waves can penetrate into the material is determined by the material’s loss tangent. The loss tangent is a measure of the material’s ability to absorb electromagnetic energy. Materials with a high loss tangent can absorb more energy and therefore heat up more quickly. Conversely, materials with a low loss tangent have a lower ability to absorb energy and therefore heat up more slowly.
Material | Loss Tangent |
---|---|
Water | 0.08 |
Food (various) | 0.1-0.6 |
Plastic | 0.005-0.02 |
Glass | 0.001-0.002 |
As shown in the table above, different materials have different loss tangents, which determines their ability to absorb energy from the electromagnetic waves. This is one of the factors that influence the heating efficiency and speed of microwave heating.
In conclusion, the skin effect and loss tangent are important concepts in understanding the physics behind microwave heating. The skin effect leads to the concentration of electromagnetic energy near the surface of the material, while the loss tangent determines the material’s ability to absorb that energy. By understanding these factors, scientists and engineers can optimize microwave heating processes for various applications.
Material Properties and Heating Efficiency
Microwave heating relies on the interaction between electromagnetic waves and the material being heated. The heating efficiency of a material depends on its properties and how well it absorbs and interacts with microwave radiation.
One important property is the dielectric constant, which measures a material’s ability to store electrical energy in an electric field. Materials with higher dielectric constants tend to absorb and convert more microwave energy into heat. This is why materials such as water, fats, and sugars heat up quickly in a microwave, as they have high dielectric constants.
Another important property is the loss tangent, which measures how well a material dissipates energy as heat. Materials with higher loss tangents, such as certain polymers, rubber, and food products, are more efficient at converting microwave energy into heat. On the other hand, materials with low loss tangents, such as glass and ceramics, are less efficient at absorbing microwave energy and may not heat up as quickly.
In addition to dielectric constant and loss tangent, the thickness and shape of a material can also affect its heating efficiency. Thinner materials, like thin films or sheets, tend to heat up more quickly than thicker materials due to the shorter distance for the microwaves to penetrate. Similarly, materials with irregular shapes or rough surfaces may experience uneven heating due to variations in the microwave absorption and reflection.
Understanding the material properties and their effects on heating efficiency is crucial for optimizing microwave heating processes. Researchers and engineers can use this knowledge to develop materials that heat up quickly and evenly, improving the efficiency and effectiveness of various applications, including cooking, heating, and material processing.
Heating Uniformity and Standing Waves
One of the challenges in microwave heating is achieving uniformity in the heating process. Microwave ovens work by emitting electromagnetic waves at a specific frequency that excite the water molecules in the food, causing them to vibrate and generate heat. However, this process can lead to uneven heating due to the formation of standing waves within the cooking chamber.
Standing waves occur when waves of the same frequency and amplitude traveling in opposite directions interfere with each other, creating regions of constructive and destructive interference. In microwave heating, these standing waves can cause hot and cold spots within the food, resulting in unevenly cooked meals.
One way to mitigate the effects of standing waves is through the use of a turntable or rotating platform in the microwave oven. By constantly rotating the food, the standing waves are disrupted, promoting more uniform heating. This is especially important for larger or bulkier items, as they are more prone to experiencing uneven heating.
Another technique to improve heating uniformity is through the use of microwave absorbers or reflectors. These materials are strategically placed within the microwave chamber to absorb or reflect the waves, thereby disrupting the formation of standing waves. By minimizing the interference patterns, the heat distribution becomes more even throughout the food.
In addition to these physical modifications, the design of the microwave oven itself can also impact heating uniformity. The placement and distribution of the magnetron (the device that generates the microwaves) and the shape and size of the cooking chamber can all influence the formation of standing waves. Manufacturers continually strive to optimize these factors to maximize the uniformity and efficiency of the heating process.
In conclusion, the phenomenon of standing waves poses a challenge to achieving heating uniformity in microwave ovens. Through the use of rotating platforms, microwave absorbers, and careful design considerations, manufacturers are constantly working to improve the efficiency and consistency of microwave heating. Understanding the physics behind standing waves is essential in unraveling the science of microwave heating and ensuring evenly cooked meals.
Nodes and Antinodes in Microwave Cavities
Nodes and antinodes play a crucial role in microwave cavities, influencing the distribution and intensity of microwave electromagnetic fields within the cavity. Understanding the properties of nodes and antinodes is important for optimizing the efficiency and effectiveness of microwave heating processes.
Nodes are points within the cavity where the microwave electromagnetic field is at a minimum. These points correspond to areas of destructive interference, where different waves of the field cancel each other out. As a result, the energy of the microwave field is concentrated elsewhere, away from the nodes.
Antinodes, on the other hand, are points within the cavity where the microwave electromagnetic field is at a maximum. These points correspond to areas of constructive interference, where different waves of the field reinforce each other. As a result, the energy of the microwave field is concentrated at the antinodes, leading to higher temperatures and more effective heating in these areas.
The distribution of nodes and antinodes within a microwave cavity depends on the shape and size of the cavity, as well as the frequency of the microwaves used. In simple rectangular or cylindrical cavities, the nodes and antinodes form regular patterns, with alternating areas of high and low field intensities.
By strategically placing the material to be heated at the antinodes of the microwave field, maximum heating efficiency can be achieved. This is why microwave ovens often have turntable plates or rotating trays, as they help to evenly distribute the heated material and expose it to different areas of the field, maximizing the chance of it being placed at an antinode.
Understanding the distribution of nodes and antinodes in microwave cavities allows for better control and optimization of microwave heating processes. By manipulating the shape and size of the cavity, as well as the frequency of the microwaves, researchers and engineers can design microwave systems with specific heating profiles, tailoring the process to the needs of different applications.
Techniques for Promoting Uniform Heating
Uniform heating is a critical factor in achieving consistent and reliable results when using microwave technology. To promote uniform heating, several techniques can be implemented:
Rotating the sample: By rotating the sample during heating, different parts of the sample will be exposed to the microwave field. This helps distribute the energy more evenly and promotes uniform heating.
Stirring or mixing: Stirring or mixing the sample ensures that all portions of the sample are exposed to the microwave field. This prevents the formation of hot spots and reduces the chances of uneven heating.
Using a turntable or rotating platform: Placing the sample on a turntable or rotating platform allows for continuous movement and ensures that all areas of the sample are evenly exposed to the microwave field. This technique is particularly useful for larger samples or samples with a higher tendency to heat unevenly.
Adding a susceptor: A susceptor is a material that absorbs microwave energy and converts it into heat. By adding a susceptor to the sample, the energy distribution can be modified, promoting more uniform heating. Susceptors can be used in various forms, such as films, coatings, or additives.
Optimizing container shape and size: The shape and size of the container holding the sample can impact the distribution of microwave energy. Using containers that have a shape that promotes even energy distribution, such as cylindrical or rectangular shapes, can help achieve uniform heating throughout the sample.
Applying insulation: Insulating the sample or its container can help control the heating rate and prevent localized overheating. Insulation materials can include microwave-safe temperature-resistant materials or specially designed microwave absorbers.
Adjusting microwave power and time: In some cases, adjusting the power level or heating time can help achieve uniform heating. By fine-tuning these parameters, hot spots can be minimized, and the overall heating distribution can be improved.
These techniques can be used individually or in combination to optimize the heating process and ensure uniform results when utilizing microwave technology. Understanding and implementing these techniques can greatly enhance the efficiency and effectiveness of microwave heating applications.
Microwave-Induced Chemical Reactions
Microwave heating has revolutionized the field of chemical reactions by providing a more efficient and precise method of heat transfer. Traditional heating methods, such as convection or radiation, can be slow and result in temperature gradients throughout the reaction vessel. In contrast, microwaves penetrate the reactant molecules directly, creating a homogeneous and rapid heating effect.
This unique heating mechanism allows for the acceleration of chemical reactions that would otherwise take hours or days to complete. The uniform heating provided by microwaves ensures that every molecule in the mixture reaches the desired temperature, promoting faster reaction rates.
Microwave-induced chemical reactions have been widely studied across various fields, including organic synthesis, materials science, and biochemistry. Researchers have discovered that different types of molecules respond differently to microwave radiation, with some exhibiting enhanced reactivity while others remain unaffected.
The specific reasons behind these differences are still the subject of ongoing research. However, several theories have been proposed to explain the microwave effect on chemical reactions. One such theory is that microwaves induce molecular vibrations, resulting in increased collision frequency and enhanced reaction rates.
Furthermore, microwaves can selectively heat polar molecules or molecules with dipole moments, leading to preferential activation of certain chemical bonds. This selectivity allows for the manipulation of reaction pathways and the synthesis of specific products that may be difficult to obtain using traditional heating methods.
In addition to promoting faster reactions and selective heating, microwaves also offer other advantages in chemical synthesis. These include improved yields, reduced side reactions, and decreased energy consumption. The ability to control temperature gradients within the reaction vessel also minimizes the formation of byproducts and increases the overall efficiency of the reaction.
Overall, microwave-induced chemical reactions have opened up new possibilities for scientists and researchers in various fields. The ability to rapidly and selectively heat reactants has broadened the scope of chemical synthesis and allowed for the development of novel materials and compounds.
Coordination and Activation Energies
In the context of microwave heating, coordination and activation energies play crucial roles in determining the efficiency and effectiveness of the process.
Coordination energy refers to the level of interaction between the microwave radiation and the molecules or particles being heated. The ability of the radiation to coordinate with the molecules affects the extent of energy transfer and the heating effect. Different materials have different coordination energies, and this can influence their response to microwave heating.
Activation energy, on the other hand, is the energy required to initiate a chemical reaction or a change in the material being heated. In the case of microwave heating, activation energy is often lower compared to conventional heating methods. This is because microwave radiation can directly excite the molecules, leading to faster and more efficient heating.
Through understanding the coordination and activation energies of different materials, scientists can optimize the microwave heating process. By selecting materials with high coordination energies and low activation energies, they can maximize energy transfer and minimize the time and energy needed for heating.
Furthermore, by studying the coordination and activation energies, researchers can also gain insights into the underlying physical processes involved in microwave heating. This knowledge can help improve the design and development of microwave heating systems and advance the overall understanding of the science behind it.
Microwave-Assisted Synthesis
Microwave-assisted synthesis is a powerful technique that has revolutionized the field of organic chemistry. By using microwave irradiation to heat reaction mixtures, this method allows for faster reaction rates and increased yields compared to traditional heating methods.
One of the key advantages of microwave-assisted synthesis is its ability to selectively heat the reaction mixture, allowing for precise control over the reaction conditions. This selective heating is due to the interaction between the microwaves and the molecules in the reaction mixture, which leads to rapid and efficient heating.
The mechanism behind microwave-assisted synthesis involves the absorption of microwave energy by polar molecules present in the reaction mixture. These polar molecules, such as water or solvents, possess dipole moments that allow them to rotate rapidly in response to the changing electric field of the microwaves.
As the polar molecules rotate, they collide with neighboring molecules, transferring their kinetic energy and effectively heating the entire reaction mixture. This rapid and localized heating results in faster reaction rates and higher yields, as the molecules are able to overcome energy barriers more easily.
Furthermore, microwave irradiation can also facilitate reactions that are not feasible under traditional heating methods. The rapid and efficient heating provided by microwaves can help overcome activation energy barriers and promote the formation of desired products.
In addition to its efficiency, microwave-assisted synthesis also offers other advantages for chemists. The technique typically requires shorter reaction times, reducing the overall time and energy required for synthesis. It also allows for greater control over reaction parameters, such as temperature and pressure, leading to enhanced selectivity and improved product quality.
Overall, microwave-assisted synthesis is a valuable tool in the chemist’s toolbox. Its ability to provide rapid and efficient heating, as well as control over reaction conditions, has made it a preferred method for various organic transformations. As the field continues to advance, further research into the physics behind microwave heating will likely uncover new opportunities for its application in synthesis.
Safety Considerations and Hazards
Microwaves are a common household appliance, but it is important to use them safely to prevent accidents and hazards. Here are some safety considerations to keep in mind:
1. Proper usage:
Read and follow the manufacturer’s instructions for your specific microwave model. This includes using microwave-safe containers and covers, and avoiding using metal or aluminum foil in the microwave, as they can cause sparks or fires.
2. Heating unevenly:
One potential hazard of microwave heating is that food might not heat evenly, creating hot spots. This can cause burns if you are not careful. Always use oven mitts or heat-resistant gloves to protect your hands when removing items from the microwave.
3. Steam and pressure buildup:
Microwaving certain foods, such as eggs or sealed containers, can lead to steam and pressure buildup. This can cause the containers to burst or explode, resulting in serious injuries. It is important to vent or open containers carefully to release steam before removing them from the microwave.
4. Chemical hazards:
Be cautious when microwaving certain liquids or foods that contain chemicals, such as oils or fatty foods. These can heat unevenly and may result in splattering or bursting, leading to burns or fires. Always use microwave-safe containers and cover them with microwave-safe lids or wraps.
5. Microwave leakage:
Although rare, an important safety consideration is microwave leakage. Microwaves can produce electromagnetic waves that can escape through damaged or poorly sealed microwave doors. To ensure safety, regularly check the seal and hinges of your microwave door and have it repaired if necessary.
Remember, microwave ovens can be convenient and efficient, but they should be used with caution and respect for the potential hazards they can pose. Following these safety considerations can help ensure a safer and more enjoyable experience with your microwave.
Leakage and Shielding
Microwave ovens are designed to contain and direct microwave energy within the cooking cavity. However, it is possible for some amount of microwave energy to leak out of the oven, which can be potentially harmful to human health and can interfere with nearby electronic devices.
To ensure the safety of the user, microwave ovens are subject to strict regulations regarding the maximum allowable amount of microwave leakage. Manufacturers are required to test every unit to ensure that it meets these safety standards. The leakage levels are typically measured in milliwatts per square centimeter (mW/cm²) at a distance of 5 centimeters from the surface of the oven.
There are several factors that can contribute to microwave leakage. One of the most common causes is a damaged or misaligned door seal. If the seal is compromised, microwave energy can escape through the gaps, leading to increased leakage. It is important to regularly inspect and clean the door seal to ensure its integrity.
Another potential source of leakage is the oven’s waveguide, which is responsible for directing the microwaves into the cavity. If the waveguide becomes corroded or damaged, it can allow microwave energy to escape. Regular inspection and maintenance of the waveguide is necessary to prevent leakage.
Shielding is another important aspect of microwave oven design. The oven cavity is typically made of metal, which acts as a shield to contain the microwaves. Additionally, the oven door is equipped with a metal mesh or screen that allows visible light to pass through but reflects or absorbs the microwaves. This mesh prevents the microwaves from escaping while still allowing the user to observe the cooking process.
In conclusion, leakage and shielding are important considerations in microwave oven design. Manufacturers must ensure that their ovens meet strict safety standards to prevent excessive microwave leakage. Regular maintenance and inspection of the door seal and waveguide are necessary to minimize leakage. The shielding provided by the metal cavity and door mesh is crucial in containing the microwaves and ensuring the safety of the user.
Thermal Runaway and Ignition
When microwaves are used to heat materials, there is always a risk of thermal runaway and ignition. Thermal runaway occurs when the heat generated by the microwaves exceeds the material’s ability to dissipate that heat, leading to a self-perpetuating cycle of increasing temperatures.
This phenomenon can be particularly dangerous when heating certain substances, such as oils and fats, as they have a low thermal conductivity and can easily reach their flash point temperature. Once a material reaches its flash point, it can ignite and start a fire.
The process of thermal runaway and ignition can be explained by the interaction between microwaves and the molecular structure of the material being heated. Microwaves oscillate at a frequency that matches the natural resonance of water molecules, causing them to rapidly vibrate and generate heat. This heat then transfers to the surrounding molecules, heating the material overall.
However, if the material’s thermal conductivity is insufficient to dissipate the generated heat, the temperature will continue to rise. This can lead to a runaway effect, where the material reaches a critical temperature that causes it to ignite and burn.
It is important to note that not all materials are susceptible to thermal runaway and ignition. Materials with higher thermal conductivity, such as metals, are less likely to experience this phenomenon. Additionally, the design and construction of microwave systems can greatly influence the potential for thermal runaway. Proper insulation and cooling mechanisms can help mitigate the risk of ignition.
In conclusion, understanding the physics behind thermal runaway and ignition is crucial when using microwaves for heating purposes. By considering the thermal properties of materials and implementing safety measures, we can effectively harness the power of microwaves while minimizing the risk of accidents and fires.
Industrial Applications of Microwave Heating
Microwave heating has found numerous industrial applications across various fields due to its ability to rapidly and uniformly heat materials. The unique properties of microwaves make them valuable tools in different sectors.
Food Industry: Microwaves have revolutionized the food industry by enabling fast and efficient cooking, heating, and drying processes. They are commonly used in microwave ovens for home and commercial cooking, eliminating the need for conventional cooking methods and reducing energy consumption. Microwaves are also utilized in food processing, such as pasteurization, sterilization, and thawing of frozen food products.
Pharmaceutical Industry: Microwave heating plays a crucial role in the pharmaceutical industry for various applications. It is extensively used for drying, sterilization, and inactivation of microorganisms during the manufacturing process. Microwaves can heat and dry pharmaceutical formulations quickly and uniformly, ensuring product quality and reducing processing time. They are also employed in the synthesis of new drug molecules.
Chemical Industry: Microwaves offer significant advantages in the chemical industry, including enhanced reaction rates, selectivity, and energy efficiency. They are employed in various chemical processes, such as polymerization, catalysis, and extraction. Microwaves enable precise and controlled heating, leading to improved product quality and reduced production costs.
Materials Processing: Microwaves are used for various materials processing applications, including sintering, brazing, and drying. They provide rapid and efficient heating, reducing processing time and energy consumption. Microwaves can also enhance the mechanical properties of materials, such as ceramic and metal, by promoting uniform heating and reducing thermal gradients.
Environmental Applications: Microwaves are utilized in environmental applications, such as soil remediation and waste treatment. They can effectively heat contaminated soil to eliminate pollutants and facilitate their removal. Microwaves are also used for the treatment of hazardous waste materials, such as medical waste, by sterilizing and deactivating pathogens.
In conclusion, microwave heating has a wide range of industrial applications, making it a versatile and valuable technology. Its ability to provide rapid, selective, and efficient heating has revolutionized various industries, improving process efficiency and product quality.
Food Processing and Sterilization
One of the key applications of microwave heating is in the field of food processing and sterilization. Microwaves offer several advantages over traditional heating methods, making them a popular choice for the food industry.
Firstly, microwave heating allows for rapid and uniform heating of food products. The microwaves penetrate the food and directly interact with water molecules present, causing them to vibrate and generate heat. This ensures that the food is heated evenly throughout, reducing the risk of cold spots or overheating.
Furthermore, microwave heating is a quick process, resulting in shorter processing times compared to conventional methods. This can lead to increased productivity and efficiency in food production facilities. It also helps in preserving the nutritional value and quality of the food, as the shorter exposure time minimizes nutrient loss and flavor changes.
In addition to heating, microwaves can also be used for sterilization purposes. The high temperatures generated by microwaves can kill microorganisms such as bacteria and viruses, making it an effective method for ensuring food safety and extending shelf life. Microwave sterilization can be particularly useful for heat-sensitive products that cannot withstand high temperatures during conventional sterilization processes.
Overall, the use of microwave heating in food processing and sterilization offers numerous benefits. It allows for rapid and uniform heating, reduces processing time, and helps preserve the nutritional value and quality of food products. Additionally, it provides an effective method for sterilization, ensuring food safety and extending shelf life. As technology continues to advance, microwave heating is likely to become even more prevalent in the food industry.